- 1Department of Dermatology, Venereology and Dermatooncology, Semmelweis University, Budapest, Hungary
- 2Queen Elizabeth Hospital, Cellular Pathology Department, University Hospitals Birmingham, Birmingham, United Kingdom
- 3Doctoral School of Economics, Business and Informatics, Corvinus University of Budapest, Budapest, Hungary
- 4Department of Health Policy, Institute of Social and Political Sciences, Corvinus University of Budapest, Budapest, Hungary
- 51st Department of Pathology and Experimental Cancer Research, Semmelweis University, Budapest, Hungary
Melanoma incidence is increasing globally. Although novel therapies have improved the survival of primary melanoma patients over the past decade, the overall survival rate for metastatic melanoma remains low. In addition to traditional prognostic factors such as Breslow thickness, ulceration, and mitotic rate, novel genetic and molecular markers have been investigated. In our study, we analyzed the expression of G-protein coupled estrogen receptor 1 (GPER1) and the endodomain of collagen XVII (COL17) in relation to clinicopathological factors in primary cutaneous melanomas with known lymph node status in both sexes, using immunohistochemistry. We found, that GPER1 expression correlated with favorable clinicopathological factors, including lower Breslow thickness, lower mitotic rate and absence of ulceration. In contrast, COL17 expression was associated with poor prognostic features, such as higher tumor thickness, higher mitotic rate, presence of ulceration and presence of regression. Melanomas positive for both GPER1 and COL17 had significantly lower mean Breslow thickness and mitotic rate compared to cases positive for COL17 only. Our data indicate that GPER1 and COL17 proteins may be of potential prognostic value in primary cutaneous melanomas.
Introduction
Melanoma is a life-threatening type of cancer that has shown a rising incidence worldwide over the past few decades [1, 2]. Major histopathological features predicting the prognosis of melanoma include Breslow thickness, ulceration, and mitotic rate [3]. In addition, age, gender, anatomical site, Clark level, lymphovascular and perineural invasion, regression, and tumor-infiltrating lymphocytes are important prognostic indicators and predictors of survival [3].
It has been observed that women exhibit a somewhat lower incidence and mortality rate of cutaneous melanoma, suggesting the role of sex hormones in tumorigenesis [4–6]. Consequently, estrogen receptors have been extensively investigated over the past decades in various malignancies, including melanoma [7–22]. Estrogen signaling and its effects are mediated through distinct receptor subtypes: ERα and ERβ, which are considered the classical estrogen receptors and function as ligand-activated transcription factors [23, 24]. Immunohistochemical and molecular studies have suggested that the downregulation of ERβ may serve as an indicator of metastatic potential in melanoma [25, 26]. Besides the classical estrogen receptors, estrogen signaling can also be mediated through the seven-transmembrane receptor, G-protein coupled estrogen receptor 1 (GPER1), which is expressed in various tissues under physiological conditions, including the nervous, reproductive, musculoskeletal systems, and gastrointestinal tract [27–30]. In tumor biology, GPER1 has been shown to facilitate cancer progression and migration, serving as a poor prognostic factor in ER-negative and human epidermal growth factor receptor 2 (HER2)-overexpressed breast and lung cancer cell lines [12–14, 19, 31, 32]. Conversely, it has also been associated with better overall survival in ER-positive and HER2-negative breast cancer [20, 32]. Furthermore, GPER1 has been reported to inhibit tumor proliferation in colorectal, prostate, and adrenocortical carcinomas, and GPER agonists have demonstrated growth inhibition in ovarian cancer [15–18, 22]. In the skin, GPER1 protein is thought to be essential for estrogen-mediated melanogenesis and is linked to hyperpigmentation during pregnancy through activation of the cAMP/pCREB/MITF signaling pathway, leading to melasma formation [33–35]. GPER1 activation inhibits melanoma proliferation in both human and murine melanoma cell lines and induces c-Myc depletion, which is associated with reduced PD-L1 expression, potentially enhancing the response to immune checkpoint inhibitor therapies [36, 37]. GPER1 knockdown has been shown to negate the effects of G-1 and tamoxifen, indicating a GPER1-dependent pathway and suggesting that GPER1 activation exerts anti-proliferative effects in melanoma [30].
In contrast to GPER1, which is associated with better outcome and mortality rates of cutaneous melanoma in women [4, 6], collagen XVII (COL17) appears to be implicated in skin cancer development [38, 39]. COL17 is a transmembrane glycoprotein of the hemidesmosome [40]. The intracellular domain of COL17 interacts with the intermediate filament network, while its extracellular domain anchors into the basement membrane zone, engaging with extracellular matrix proteins [41]. Beyond its structural role in the hemidesmosome complex, overexpression of COL17 is presumed to play a role in cancer development, tumor invasion, and decreased survival rates in squamous cell carcinoma, as well as colorectal, lung and pancreatic cancer [39, 42–47]. Contrarily, in human breast cancer, COL17 expression has been found to be decreased [48]. Krenács et al. showed that the cell-residual 60 kDa endodomain of COL17 (but not the shedding ectodomain) can be detected in primary and metastatic human melanoma as well as melanoma cell lines, but not in resting melanocytes and nevi [49].
As stated above, the existing literature has reported on the expression of GPER1 and COL17 in various tumors, including melanoma, using immunohistochemistry and mRNA levels, yet without specific emphasis on lymph node metastatic status. In our retrospective study, we aimed to investigate the expression of GPER1 and the cell-residual endodomain of COL17 proteins in primary melanoma samples with known lymph node status in relation to the major clinicopathological factors.
Materials and methods
Study population
Ethics approval was obtained from the Semmelweis University Institutional Review Board (32-4/2007). The database of the Department of Dermatology, Venereology and Dermatooncology of Semmelweis University was reviewed for melanoma patients who were diagnosed between 1st of January 2000, and 31st of December 2010, and completed a 10-year follow-up period. The total number of patients (n = 94) had an equal distribution of males (n = 47) and females (n = 47, respectively). The female patients were further subcategorized as premenopausal (<45 years old) and postmenopausal (≥45 years old) [50]. The registered clinicopathological characteristics are listed in Table 1. All examined melanoma samples were grouped according to the Breslow thickness categories reported in the 2018 American Joint Committee on Cancer (AJCC) melanoma staging system [51].
Immunohistochemistry
The protein expressions of GPER1 and COL17 were evaluated in archived, formalin-fixed, paraffin-embedded melanoma tissue samples from primary melanomas of the 94 patients. Three µm thick sections were cut, dewaxed in xylene and rehydrated in decreasing concentrations of ethanol. Heat-induced epitope retrieval was done by boiling slides for 20 min in 500 mL of 0.1 M Tris-buffered-saline (TBS) containing 0.01 M ethylenediamine-tetraacetic acid (Tris-EDTA), pH 9.0, followed by 20 min cooling with open lid. The blocking of endogenous peroxidases was completed in 3% hydrogen peroxide (H2O2) diluted in methanol for 20 min, followed by blocking of non-specific antigen binding in TBS, pH 7.4, for 30 min containing 3% bovine serum albumin (BSA, #82-100-6, Millipore, Kankakee, Illinois, United States). For the detection of target proteins, rabbit polyclonal GPER1 (#NLS4271, 1:100; GPER/GPR30, Bio-Techne, Abingdon, United Kingdom) and mouse monoclonal anti-COL17 antibody clone 9G2, recognizing the aa507–529 uppermost extracellular part of COL17a endodomain region, as produced, validated and described by Stelkovics et al. [42, 49] antibodies were applied overnight in a humidified chamber. Tissue-bound antibody detection was performed with MACH4 Universal HRP-polymer, biotin-free detection (Biocare Medical, Concord, MA, United States). Aminoethyl-carbazole (AEC) H2O2 chromogen-substrate system dissolved in 0.1 M acetate buffer, pH 4.6, was used for revealing the peroxidase activity under microscopic control followed by nuclear staining using hematoxylin. After mounting, stained slides were digitalized using Panoramic Scan (3DHISTECH, Budapest, Hungary).
Assessment of immunostaining
Single immunolabeling for GPER1 and COL17 was completed on serial sections from each sample. Immunohistochemical scoring for both proteins was completed using the Histo-score (H-score), incorporating both the staining intensity and a percentage of stained tumor cells at each intensity level. The staining intensity values were indicated as 0 (absent), 1 (mild staining), 2 (moderate staining), and 3 (intensive staining) (Figure 1), which were then multiplied with the percentage of stained cells in the sample (ranging from 0% to 100%). The final H-score is derived from the sum of staining intensity values multiplied by percentage of stained cells as the equation shown. This score, therefore, is in the range of 0–300 (H-score = [0 × (% cells 0) + 1 × (% cells 1+) + 2 × (% cells 2+) + 3 × (% cells 3+)]) [52–55].
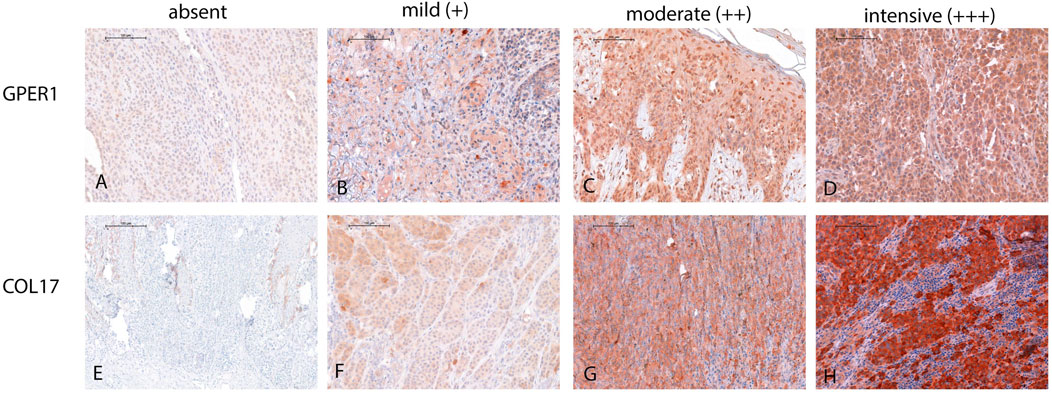
Figure 1. Illustration of GPER1 and COL17 protein expression intensity in the four-tier system in melanoma. (A) Representative image demonstrating the absence of GPER1 labelling. (B–D) Intensity differences between GPER1 positive tumor cells. (E) Absence of COL17 labelling of the tumor cells, only healthy keratinocytes at the basal layer showed positivity for COL17. (F–H) Intensity differences between COL17 positive tumor cells (for all images: original magnification ×40, scale bar: 100 µm).
In order to minimize potential bias, the scoring was independently performed by four investigators (referred to as UC, MF, PB, TK). Disagreements in scoring were resolved through discussion and mutual consensus. Breast cancer tissue microarray (TMA) samples served as positive external controls for GPER1. As internal positive control of GPER1 staining, keratinocytes as well as sebaceous and sweat gland epithelia were used. For COL17, the surrounding healthy skin in each sample was deemed as positive control. Omitting the primary antibodies in each run served as negative controls.
Statistical analysis
First, descriptive statistics were calculated for all variables. Then bivariate analyses were conducted, non-parametric Mann-Whitney U test was performed between groups and Pearson correlation analysis was used to determine the strength of the association between continuous variables, while cross tabulation analysis with Spearman’s chi-squared tests was performed between categorical variables. Statistical analyses were carried out by IBM SPSS Statistics for Windows, Version 25.0. Armonk, NY: IBM Corp.
Results
GPER1 protein expression and clinicopathological factors of melanoma
GPER1 expression was detected in over half of the melanoma samples (n = 54/94, 57.45%). The majority of thinner melanomas [Breslow thickness ≤2 mm (n = 40/57, 70.18%)] were positive for GPER1.
GPER1 positive melanoma samples had significantly lower Breslow thickness (p = 0.01) and mitotic rate (p = 0.007) when compared to GPER1 negative cases (Figure 2). Mean H-score of GPER1 positive cases with Breslow thickness of more than 2 mm (H-score: 47.69) was significantly lower when compared with melanoma samples with Breslow thickness of 2 mm and less (H-score: 94.90) (p < 0.005). Furthermore, the presence of GPER1 receptor positivity showed inverse correlation with the presence of ulceration and sentinel lymph node (SLN) positivity based on cross-tabulation analyses (Table 2).
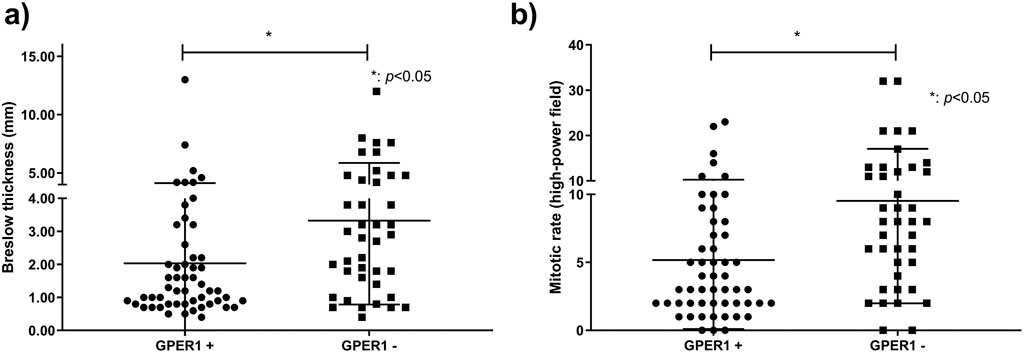
Figure 2. Association of Breslow thickness and mitotic rate with GPER1 protein expression. Dots and squares represent Breslow thickness (n = 94) (A) and mitotic rate (n = 93) (B) of individual primary melanomas.
With regards to the different subtypes of melanoma, GPER1 protein was expressed in the majority of superficial spreading melanomas (SSM, n = 50/83, 60.20%), while it was only seen in one case out of the five nodular melanomas (NM) (Supplementary Table S1).
Considering the gender difference, GPER1 protein expression was more frequently detected in females compared to males (n = 31/47 versus n = 23/47, p = 0.095) (Supplementary Table S1).
GPER1 expression showed no statistically significant relationship with peritumoral lymphocytic infiltrate (PLI), lymph node or distant metastases, patient’s age or between pre- and postmenopausal women group (Supplementary Table S1).
COL17 endodomain expression and clinicopathological factors of melanoma
COL17 endodomain expression was found in nearly two-third of the melanoma samples (n = 62/94, 65.96%), and was predominantly present along the growing tumor front (Figure 3). The majority of thicker melanomas [Breslow thickness >2 mm (n = 32/37, 86.48%)] were positive for COL17 endodomain.
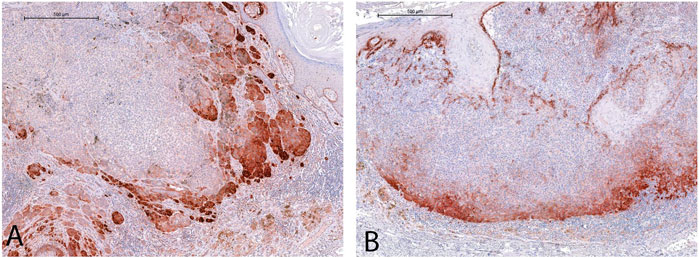
Figure 3. Cytoplasmic COL17 protein expression was mostly observed along the lateral (A) and deep (B), growing fronts of the tumors. [See endogenous positive control reaction in the basal epidermal layer in (B)] (For both images: original magnification ×5, scale bar: 500 µm).
COL17 endodomain expression showed positive correlation with increased Breslow thickness (p = 0.004) and mitotic rate (p = 0.0009) (Figure 4). Mean H-score of COL17 endodomain positive cases with Breslow thickness of more than 2 mm (H-score: 77.44) was significantly higher than cases with Breslow thickness of 2 mm and less (H-score: 29.81) (p < 0.001). Furthermore, COL17 endodomain-expressing tumors exhibited more frequently unfavorable characteristics such as ulceration, regression, and SLN positivity (Table 3).
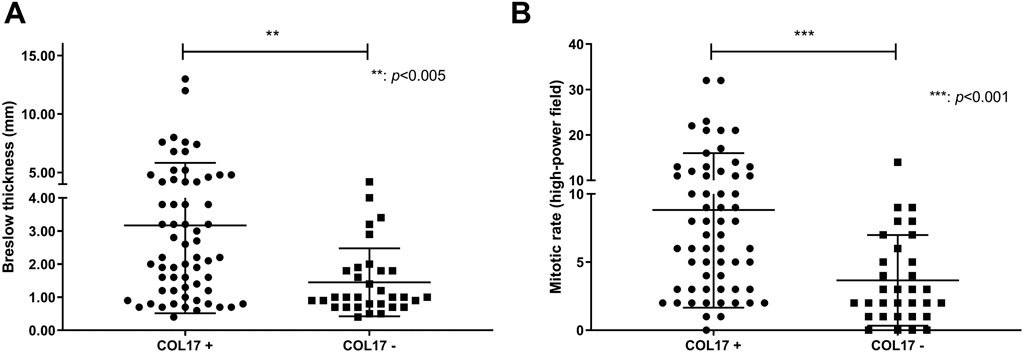
Figure 4. Association of Breslow thickness and mitotic rate with COL17 protein expression. Dots and squares represent Breslow thickness (n = 94) (A) and mitotic rate (n = 93) (B) of individual primary melanomas.
The presence of distant lymph node and cutaneous/visceral metastases was more commonly seen in samples with COL17 endodomain expression, compared to COL17 negative tumors (Supplementary Table S2). The expression of the protein was observed more frequently in melanomas located on the trunk (n = 37/53, 69.81%) and upper extremities (n = 10/13, 76.92%) than in those with localization along the lower extremities (n = 13/28, 46.43%) (Supplementary Table S2).
Considering the histological subtypes, almost all nodular melanoma cases (n = 4/5, 80.00%) showed COL17 endodomain expression, and a subset of superficial spreading melanomas were also positive for COL17 endodomain (54/83, 65.00%) (Supplementary Table S2).
COL17 endodomain expression showed no significant association with gender, age, or presence of PLI (Supplementary Table S2).
GPER1 and COL17 double expression
Nearly one-third (n = 32/94, 34.04%) of the cases exhibited simultaneous positivity for GPER1 and COL17 proteins. Melanomas positive for both markers had significantly lower mean Breslow thickness (2.425 mm vs. 3.957 mm, p = 0.004) and mitotic rate (6.61/HPF vs. 11.1/HPF, p = 0.008) compared to cases positive for COL17 only (Table 4). Conversely, double-positive cases had higher mean Breslow thickness (2.425 mm vs. 1.464 mm, p = 0.056) and mitotic rate (6.61/HPF vs. 3.14/HPF, p = 0.003) compared to cases positive for GPER1 only (Table 4).
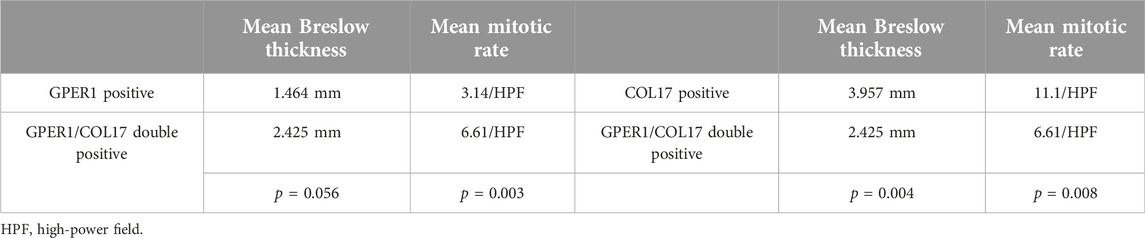
Table 4. Comparison of mean Breslow thickness and mean mitotic rate of GPER1/COL17 double positive cases (n = 32) with GPER1 (n = 22) or COL17 (n = 30) positive cases.
Discussion
In this retrospective study, we aimed to explore the expression patterns of GPER1 and the COL17 endodomain proteins in primary cutaneous melanoma tissue samples from patients with known sentinel lymph node status and correlated this with the common clinicopathological features of melanoma.
GPER1 protein expression has been described in various tissues under physiological conditions including the nervous-, reproductive-, musculoskeletal system, and gastrointestinal tract [27–29]. Human and animal studies both revealed the role of GPER1 in the regulation of physiological responses involving mammary gland development [56], oocyte maturation [57, 58], endometrial cell growth [59], cardiomyocyte growth [60], vasodilation [61, 62], T-cell differentiation [63], inhibition of inflammation [64, 65], insulin secretion [61, 62], and chondrocyte differentiation [66]. In the murine skin, GPER1 mediates melanocyte differentiation and melanin pigment production; and it has also been shown to decreases expression of the oncodriver c-Myc, as described by Natale et al. using murine melanoma xenograft models [37].
Besides its expression in normal tissues, GPER1 has also been found in many types of cancers/cancer cell lines. Its role has been most intensively studied in breast cancer, where it appears to contribute to cancer cell proliferation, migration and invasion through its ability to transactivate epidermal growth factor receptors (EGFRs) [14, 67]. However, it was also shown to be a predictor of better overall survival (OS) in ER-positive breast cancer [20]. In ovarian cancer cells, GPER1 can be detected both within the nucleus and the cytoplasm, and the nuclear GPER1 is a potential predictive factor of poor survival [68]. Similar to its effect in breast cancer, GPER1 promotes cell proliferation, migration, lymph node metastasis and invasion in ER-negative ovarian cancer, and its overexpression correlates with tumor size and stage [69–71]. Cytoplasmic GPER1 overexpression was also found in high-grade estrogen receptor- and progesterone receptor-negative endometrial adenocarcinomas in association with myometrial invasion and poor survival [72]. Furthermore, GPER1 agonists enhance tumor growth of endometrial cancer cell line xenografts [73]. Similar to gynecological cancers, GPER1 (over) expression in lung cancer is also associated with high TNM stage and lymph node metastasis [13, 15, 74]. In human non-small-cell lung cancer cell lines, the expression of cytoplasmic GPER1 was high [13] and treatment of non-small-cell lung cancer cell lines with GPER1 antagonists impaired tumor growth [75]. In colorectal cancer, on the other hand, GPER1 appears to act as a tumor suppressor and its expression is negatively correlated with increased tumor stage and lymph node metastasis [16]. Moreover, higher GPER1 expression meant survival benefit for colorectal cancer patients [16]. In adrenocortical cancer, GPER1 presented tumor suppressive properties as GPER1 agonists suppressed adrenocortical carcinoma proliferation via cell cycle arrest, DNA damage, and apoptosis via ERK1/2 activation [18].
In our previous study [6], we found that GPER1 expression in pregnancy-related melanoma samples was associated with lower Breslow thickness, lower mitotic rate, lower hazard of local or distant metastases, and the protein expression was inversely associated with the presence of ulceration [6].
Our current study cohort, which included both genders and known sentinel lymph node status for all patients, showed similar results. GPER1 protein expression was correlated with lower Breslow thickness and mitotic rate. The presence of ulceration and sentinel lymph node metastases were found less frequently in GPER1-positive cases, suggesting that GPER1 may serve as a favorable prognostic marker.
Considering the role of GPER1 in cancer pathophysiology, it has been suggested as a potential target for cancer therapy [30]. In vivo and in vitro studies using GPER1 agonists such as G-1 demonstrated beneficial effects for cancer prognosis; these involved the inhibition of proliferation and the promotion of apoptosis in leukemia cell lines of T lineage [76], as well as the inhibition of growth in both mantle cell lymphoma [77] and pancreatic ductal adenocarcinoma [78]. Furthermore, G-1 assisted temozolamide to impair glioblastoma proliferation [79], and inhibited the growth of ovarian cancer cells [80] and gastric cancer [81]. In melanoma, the activation of GPER1 by its specific agonist G-1, inhibited the proliferation of mouse melanoma cell lines by decreasing cell division and blocking cell cycle progression in the G2 phase [36]. In a murine model, co-treatment with PD-1 inhibitor and GPER1 agonist G-1 resulted in reduced cell proliferation and tumor volume along with better survival [37]. In vitro treatment of uveal melanoma cell lines with GPER1 agonist induced mitotic arrest and apoptosis of tumor cells [82]. Based on these observations, an ongoing phase I clinical trial has been initiated using pembrolizumab and a selective agonist of GPER1 for treatment of melanoma [83].
After establishing the favorable prognostic aspects of GPER1, we sought to identify a marker that could potentially have reverse effects in our cohort. Based on our research group’s previous findings, namely, that uppermost extracellular part (aa507-529) of COL17a endodomain (but not the shedding ectodomain) is expressed in proliferating melanocytes and melanomas but not in benign melanocytic lesions [49], we aimed to examine COL17 expression in our current primary cutaneous melanoma cohort. The overexpression of COL17 coding mRNA and protein levels in colorectal carcinoma was associated with higher TNM staging, infiltrative growth, metastases, and decreased survival rates [45]. In lung cancer, elevated expression of COL17 was seen in the stromal environment and was associated with increased metastatic potential [47]. Furthermore, COL17 may contribute to the epithelial-to-mesenchymal transition and poor disease prognosis [84]. Overexpression of COL17 in cervix carcinoma exhibited a relation to increased local dissemination and metastasis [85, 86]. In contrast, COL17 showed reduced expression in advanced breast cancer [48] in correlation with higher TNM staging, increased invasion, postmenopausal status and poorer prognosis [48]. The antagonistic expression of COL17 in breast cancer is suggested to be due to hypermethylation of the COL17 coding gene promoter [86]. The role of COL17 was widely studied in both normal skin and cutaneous malignancies [39, 42, 43], also in head and neck squamous cell carcinoma (SCC) [87, 88]. SCC demonstrated COL17 overexpression promoting tumor invasion [42, 89]. Krenács et al. found that the cell residual endodomain, COL17, but not the ectodomain, was expressed in melanoma, but not in normal melanocytic and dysplastic nevi. It was also shown that immunological targeting of this protein sequence of COL17 with a specific antibody lead to apoptosis in melanoma cell lines [49]. Another study using murine melanoma models demonstrated that dysfunction of COL17 in keratinocytes promoted melanoma progression [90]. A recent publication demonstrated that in mucosal melanoma, the COL17 coding gene has a variant (p.Ser1029Ala) in the ectodomain, which may be less efficiently shed compared to the wild-type, thereby assisting melanoma progression [91]. All these previous data suggested the dysfunction of COL17 and its potential role in melanoma development and progression.
The current study shows that in primary melanoma samples, the expression of COL17 endodomain had an inverse relationship when compared to GPER1 expression by showing positive association with poor prognostic factors including increased Breslow thickness, higher mitotic rate and presence of ulceration. COL17 endodomain expression was more frequently seen in sentinel lymph node positive melanomas. Furthermore, the overexpression of COL17 along the invasive tumor front was suggestive of its potential role in tumor invasion in line with some earlier results: for example, COL17 was found to be at the invasive tumor fronts in squamous cell carcinoma of the tongue [89].
While there has been no direct interactions described so far between GPER1 and COL17 at a molecular level, COL17 protein has already been shown to inhibit breast cancer cell proliferation and growth via decreasing the phosphorylation of key proteins in the AKT/mTOR pathway [92], which is known to be one of the major downstream signaling target of the GPER1 pathway as well [93–96]. GPER1, in reverse, is found to enhance the phosphorylation of AKT/mTOR pathway leading to its activation in breast, ovarian and lung cancer resulting in increased cell proliferation in these types of cancers [93–95].
The rationale for investigating both GPER1 and COL17 concurrently was to determine whether their co-expression shows any correlation with the major clinicopathological factors of melanoma. Co-expression of both proteins in our study was observed in nearly one-third of the cases. Melanomas positive for both GPER1 and COL17 exhibited lower mean Breslow thickness and mitotic rates compared to cases positive for COL17 alone. Conversely, double-positive cases demonstrated higher mean Breslow thickness and mitotic rates than cases positive for GPER1 alone. Theoretically, while GPER1 expression decreases with increasing tumor size, COL17 expression emerges in high-risk, thicker melanomas, particularly at deeper invasive fronts. Our current results suggest that double positivity for GPER1 and COL17 may indicate an intermediate phase in tumor progression, however, future studies with larger cohorts and detailed molecular analyses are necessary to study any potential link between the two proteins and their potential interactions.
Although the association of GPER1 with favorable prognostic clinicopathological features suggests a trend towards better overall survival (OS), and COL17 endodomain is anticipated to correlate with poorer OS, a larger cohort of patients will be necessary in future studies to substantiate these findings.
In summary, our study demonstrated that GPER1 expression is associated with lower Breslow thickness, lower mitotic rate, absence of ulceration, and absence of sentinel lymph node metastasis in both genders, all of which are predictive clinicopathological factors for better survival outcomes. In contrast, COL17 endodomain expression in human melanoma, when present along the growing tumor front, suggests its involvement in melanoma invasion. COL17 endodomain expression was proven to be associated with poor prognostic features such as greater tumor thickness, higher mitotic rate, presence of regression, and sentinel lymph node positivity, indicating worse survival outcomes. Our findings suggest that the immunohistochemical detection of GPER1 and COL17 proteins in melanoma may serve as valuable prognostic markers.
Limitations of our study include the relatively small patient cohort, the retrospective study design, and the exclusive use of immunohistochemistry for biomarker detection. Further investigations with larger patient cohorts, supplemented by functional experiments, are necessary to corroborate our findings and elucidate the potential role of GPER1 and COL17 protein expression in melanoma.
Data availability statement
The raw data supporting the conclusions of this article will be made available by the authors, without undue reservation.
Ethics statement
The studies involving humans were approved by the Semmelweis University Institutional Review Board. The studies were conducted in accordance with the local legislation and institutional requirements. Written informed consent for participation was not required from the participants or the participants’ legal guardians/next of kin in accordance with the national legislation and institutional requirements.
Author contributions
UC, MF, PB, and TK planned the experiments, UC conducted the experiments. UC, MF, PB, and TK scored the immunohistochemistry stainings. AF and VB conducted the statistical analysis. All authors contributed to the article and approved the submitted version.
Funding
The authors declare that financial support was received for the research, authorship, and/or publication of this article. This work was supported by the Nékám Foundation and by the Hungarian Scientific Research Fund (OTKA NN 114460).
Acknowledgments
The authors are grateful to Krisztián Németh for his help in editing the manuscript. We are thankful to Judit Hársing and Enikő Kuroli for their help with gathering paraffin-based melanoma tissue sections from the histopathology archive of the Department of Dermatology, Venereology and Dermatooncology, as well as for their help with hematoxylin-eosin staining of the tissue samples. We thank to Lajos Vince Kemény, Zsófia Csábi and Benedek Nagy for their help with literature analysis.
Conflict of interest
The authors declare that the research was conducted in the absence of any commercial or financial relationships that could be construed as a potential conflict of interest.
Supplementary material
The Supplementary Material for this article can be found online at: https://www.por-journal.com/articles/10.3389/pore.2024.1611809/full#supplementary-material
References
1. Erdmann, F, Lortet-Tieulent, J, Schüz, J, Zeeb, H, Greinert, R, Breitbart, EW, et al. International trends in the incidence of malignant melanoma 1953–2008--are recent generations at higher or lower risk? Int J Cancer (2013) 132:385–400. doi:10.1002/ijc.27616
2. Sacchetto, L, Zanetti, R, Comber, H, Bouchardy, C, Brewster, DH, Broganelli, P, et al. Trends in incidence of thick, thin and in situ melanoma in Europe. Eur J Cancer Oxf Engl (2018) 92:108–18. doi:10.1016/j.ejca.2017.12.024
3. Current Staging. Prognostic factors in melanoma- ClinicalKey. Available from: https://www.clinicalkey.com/#!/content/playContent/1-s2.0-S1055320714001240?returnurl=https:%2F%2Flinkinghub.elsevier.com%2Fretrieve%2Fpii%2FS1055320714001240%3Fshowall%3Dtrue&referrer=https:%2F%2Fpubmed.ncbi.nlm.nih.gov%2F (Accessed March 9, 2021).
4. Bellenghi, M, Puglisi, R, Pontecorvi, G, De Feo, A, Carè, A, and Mattia, G. Sex and gender disparities in melanoma. Cancers (2020) 12:1819. doi:10.3390/cancers12071819
5. Smalley, KS. Why do women with melanoma do better than men? eLife (2018) 7:e33511. doi:10.7554/eLife.33511
6. Fábián, M, Rencz, F, Krenács, T, Brodszky, V, Hársing, J, Németh, K, et al. Expression of G protein-coupled oestrogen receptor in melanoma and in pregnancy-associated melanoma. J Eur Acad Dermatol Venereol JEADV (2017) 31:1453–61. doi:10.1111/jdv.14304
7. Dika, E, Patrizi, A, Lambertini, M, Manuelpillai, N, Fiorentino, M, Altimari, A, et al. Estrogen receptors and melanoma: a Review. Cells (2019) 8:1463. doi:10.3390/cells8111463
8. Zhou, JH, Kim, KB, Myers, JN, Fox, PS, Ning, J, Bassett, RL, et al. Immunohistochemical expression of hormone receptors in melanoma of pregnant women, nonpregnant women, and men. Am J Dermatopathol (2014) 36:74–9. doi:10.1097/DAD.0b013e3182914c64
9. Dika, E, Fanti, PA, Vaccari, S, Capizzi, E, Degiovanni, A, Gobbi, A, et al. Oestrogen and progesterone receptors in melanoma and nevi: an immunohistochemical study. Eur J Dermatol EJD (2017) 27:254–9. doi:10.1684/ejd.2017.3019
10. de Giorgi, V, Mavilia, C, Massi, D, Sestini, S, Grazzini, M, Brandi, ML, et al. The role of estrogens in melanoma and skin cancer. Carcinogenesis (2009) 30:720. doi:10.1093/carcin/bgp025
11. Lee, HJ, Wall, B, and Chen, S. G-protein-coupled receptors and melanoma. Pigment Cell Melanoma Res (2008) 21:415–28. doi:10.1111/j.1755-148X.2008.00478.x
12. Gründker, C, and Emons, G. The role of gonadotropin-releasing hormone in cancer cell proliferation and metastasis. Front Endocrinol (2017) 8:187. doi:10.3389/fendo.2017.00187
13. Jala, VR, Radde, BN, Haribabu, B, and Klinge, CM. Enhanced expression of G-protein coupled estrogen receptor (GPER/GPR30) in lung cancer. BMC Cancer (2012) 12:624. doi:10.1186/1471-2407-12-624
14. Meng, R, Qin, Q, Xiong, Y, Wang, Y, Zheng, J, Zhao, Y, et al. NHERF1, a novel GPER associated protein, increases stability and activation of GPER in ER-positive breast cancer. Oncotarget (2016) 7:54983–97. doi:10.18632/oncotarget.10713
15. Jacenik, D, Cygankiewicz, AI, and Krajewska, WM. The G protein-coupled estrogen receptor as a modulator of neoplastic transformation. Mol Cell Endocrinol (2016) 429:10–8. doi:10.1016/j.mce.2016.04.011
16. Liu, Q, Chen, Z, Jiang, G, Zhou, Y, Yang, X, Huang, H, et al. Epigenetic down regulation of G protein-coupled estrogen receptor (GPER) functions as a tumor suppressor in colorectal cancer. Mol Cancer (2017) 16:87. doi:10.1186/s12943-017-0654-3
17. Lau, K-M, Ma, FM-T, Xia, JT, Chan, QKY, Ng, CF, and To, KF. Activation of GPR30 stimulates GTP-binding of Gαi1 protein to sustain activation of Erk1/2 in inhibition of prostate cancer cell growth and modulates metastatic properties. Exp Cell Res (2017) 350:199–209. doi:10.1016/j.yexcr.2016.11.022
18. Chimento, A, Sirianni, R, Casaburi, I, Zolea, F, Rizza, P, Avena, P, et al. GPER agonist G-1 decreases adrenocortical carcinoma (ACC) cell growth in vitro and in vivo. Oncotarget (2015) 6:19190–203. doi:10.18632/oncotarget.4241
19. Talia, M, De Francesco, EM, Rigiracciolo, DC, Muoio, MG, Muglia, L, Belfiore, A, et al. The G protein-coupled estrogen receptor (GPER) expression correlates with pro-metastatic pathways in ER-negative breast cancer: a bioinformatics analysis. Cells (2020) 9:622. doi:10.3390/cells9030622
20. Zhang, D, Wang, J, Chen, H, and Yan, S. Cytoplasmic G protein-coupled estrogen receptor 1 as a prognostic indicator of breast cancer: a meta-analysis. Technol Cancer Res Treat (2022) 21:15330338221131664. doi:10.1177/15330338221131664
21. Martin, SG, Lebot, MN, Sukkarn, B, Ball, G, Green, AR, Rakha, EA, et al. Low expression of G protein-coupled oestrogen receptor 1 (GPER) is associated with adverse survival of breast cancer patients. Oncotarget (2018) 9:25946–56. doi:10.18632/oncotarget.25408
22. Lv, X, Hua, G, He, C, Davis, JS, and Wang, C. Abstract B82: G-protein coupled estrogen receptor (GPER) agonist G-1 inhibits growth of human granulosa cell tumor cells via blocking microtubule assembly. Clin Cancer Res (2014) 19:B82. doi:10.1158/1078-0432.OVCA13-B82
23. Glatthaar, H, Katto, J, Vogt, T, and Mahlknecht, U. Estrogen receptor alpha (ESR1) single-nucleotide polymorphisms (SNPs) affect malignant melanoma susceptibility and disease course. Genet Epigenetics (2016) 8:1–6. doi:10.4137/GEG.S31264
24. Fuentes, N, and Silveyra, P. Estrogen receptor signaling mechanisms. Adv Protein Chem Struct Biol (2019) 116:135–70. doi:10.1016/bs.apcsb.2019.01.001
25. de Giorgi, V, Mavilia, C, Massi, D, Gozzini, A, Aragona, P, Tanini, A, et al. Estrogen receptor expression in cutaneous melanoma: a real-time reverse transcriptase-polymerase chain reaction and immunohistochemical study. Arch Dermatol (2009) 145:30–6. doi:10.1001/archdermatol.2008.537
26. Marzagalli, M, Montagnani Marelli, M, Casati, L, Fontana, F, Moretti, RM, and Limonta, P. Estrogen receptor β in melanoma: from molecular insights to potential clinical utility. Front Endocrinol (2016) 7:140. doi:10.3389/fendo.2016.00140
27. Xu, S, Yu, S, Dong, D, and Lee, LTO. G protein-coupled estrogen receptor: a potential therapeutic target in cancer. Front Endocrinol (2019) 10:725. doi:10.3389/fendo.2019.00725
28. Kvingedal, AM, and Smeland, EB. A novel putative G-protein-coupled receptor expressed in lung, heart and lymphoid tissue. FEBS Lett (1997) 407:59–62. doi:10.1016/s0014-5793(97)00278-0
29. Feng, Y, and Gregor, P. Cloning of a novel member of the G protein-coupled receptor family related to peptide receptors. Biochem Biophys Res Commun (1997) 231:651–4. doi:10.1006/bbrc.1997.6161
30. Pepermans, RA, Sharma, G, and Prossnitz, ER. G protein-coupled estrogen receptor in cancer and stromal cells: functions and novel therapeutic perspectives. Cells (2021) 10:672. doi:10.3390/cells10030672
31. Sjöström, M, Hartman, L, Fornander, T, Grabau, D, Malmström, P, Nordenskjöld, B, et al. Abstract P1-08-12: G protein-coupled estrogen receptor in the plasma membrane is prognostic in early breast cancer. Cancer Res (2013) 73:P1–08-12-P1-08-12. doi:10.1158/0008-5472.SABCS13-P1-08-12
32. Yang, F, and Shao, Z-M. Double-edged role of G protein-coupled estrogen receptor 1 in breast cancer prognosis: an analysis of 167 breast cancer samples and online data sets. Oncotargets Ther (2016) 9:6407–15. doi:10.2147/OTT.S111846
33. Natale, CA, Duperret, EK, Zhang, J, Sadeghi, R, Dahal, A, O'Brien, KT, et al. Sex steroids regulate skin pigmentation through nonclassical membrane-bound receptors. eLife (2016) 5:e15104. doi:10.7554/eLife.15104
34. Nicolaidou, E, and Katsambas, AD. Pigmentation disorders: hyperpigmentation and hypopigmentation. Clin Dermatol (2014) 32:66–72. doi:10.1016/j.clindermatol.2013.05.026
35. Sun, M, Xie, H-F, Tang, Y, Lin, SQ, Li, JM, Sun, SN, et al. G protein-coupled estrogen receptor enhances melanogenesis via cAMP-protein kinase (PKA) by upregulating microphthalmia-related transcription factor-tyrosinase in melanoma. J Steroid Biochem Mol Biol (2017) 165:236–46. doi:10.1016/j.jsbmb.2016.06.012
36. Ribeiro, MPC, Santos, AE, and Custódio, JBA. The activation of the G protein-coupled estrogen receptor (GPER) inhibits the proliferation of mouse melanoma K1735-M2 cells. Chem Biol Interact (2017) 277:176–84. doi:10.1016/j.cbi.2017.09.017
37. Natale, CA, Li, J, Zhang, J, Dahal, A, Dentchev, T, Stanger, BZ, et al. Activation of G protein-coupled estrogen receptor signaling inhibits melanoma and improves response to immune checkpoint blockade. eLife (2018) 7:e31770. doi:10.7554/eLife.31770
38. Mallipeddi, R, Keane, FM, McGrath, JA, Mayou, BJ, and Eady, RAJ. Increased risk of squamous cell carcinoma in junctional epidermolysis bullosa. J Eur Acad Dermatol Venereol JEADV (2004) 18:521–6. doi:10.1111/j.1468-3083.2004.00968.x
39. Jones, VA, Patel, PM, Gibson, FT, Cordova, A, and Amber, KT. The role of collagen XVII in cancer: squamous cell carcinoma and beyond. Front Oncol (2020) 10:352. doi:10.3389/fonc.2020.00352
40. Hammers, CM, and Stanley, JR. Mechanisms of disease: pemphigus and bullous pemphigoid. Annu Rev Pathol (2016) 11:175–97. doi:10.1146/annurev-pathol-012615-044313
41. Koster, J, Geerts, D, Favre, B, Borradori, L, and Sonnenberg, A. Analysis of the interactions between BP180, BP230, plectin and the integrin alpha6beta4 important for hemidesmosome assembly. J Cell Sci (2003) 116:387–99. doi:10.1242/jcs.00241
42. Stelkovics, E, Korom, I, Marczinovits, I, Molnar, J, Rasky, K, Raso, E, et al. Collagen XVII/BP180 protein expression in squamous cell carcinoma of the skin detected with novel monoclonal antibodies in archived tissues using tissue microarrays and digital microscopy. Appl Immunohistochem Mol Morphol AIMM (2008) 16:433–41. doi:10.1097/PAI.0b013e318162f8aa
43. Moilanen, JM, Löffek, S, Kokkonen, N, Salo, S, Väyrynen, JP, Hurskainen, T, et al. Significant role of collagen XVII and integrin β4 in migration and invasion of the less aggressive squamous cell carcinoma cells. Sci Rep (2017) 7:45057. doi:10.1038/srep45057
44. Meng, X, Matsumoto, F, Mori, T, Miura, N, Ino, Y, Onidani, K, et al. BP180 is a prognostic factor in head and neck squamous cell carcinoma. Anticancer Res (2021) 41:1089–99. doi:10.21873/anticanres.14867
45. Moilanen, JM, Kokkonen, N, Löffek, S, Väyrynen, JP, Syväniemi, E, Hurskainen, T, et al. Collagen XVII expression correlates with the invasion and metastasis of colorectal cancer. Hum Pathol (2015) 46:434–42. doi:10.1016/j.humpath.2014.11.020
46. Laval, S, Laklai, H, Fanjul, M, Pucelle, M, Laurell, H, Billon-Galés, A, et al. Dual roles of hemidesmosomal proteins in the pancreatic epithelium: the phosphoinositide 3-kinase decides. Oncogene (2014) 33:1934–44. doi:10.1038/onc.2013.146
47. Papay, J, Krenacs, T, Moldvay, J, Stelkovics, E, Furak, J, Molnar, B, et al. Immunophenotypic profiling of nonsmall cell lung cancer progression using the tissue microarray approach. Appl Immunohistochem Mol Morphol AIMM (2007) 15:19–30. doi:10.1097/01.pai.0000213143.32030.f5
48. Bergstraesser, LM, Srinivasan, G, Jones, JC, Stahl, S, and Weitzman, SA. Expression of hemidesmosomes and component proteins is lost by invasive breast cancer cells. Am J Pathol (1995) 147:1823–39.
49. Krenacs, T, Kiszner, G, Stelkovics, E, Balla, P, Teleki, I, Nemeth, I, et al. Collagen XVII is expressed in malignant but not in benign melanocytic tumors and it can mediate antibody induced melanoma apoptosis. Histochem Cell Biol (2012) 138:653–67. doi:10.1007/s00418-012-0981-9
50. Blondeaux, E, Massarotti, C, Fontana, V, Poggio, F, Arecco, L, Fregatti, P, et al. The PREgnancy and FERtility (PREFER) study investigating the need for ovarian function and/or fertility preservation strategies in premenopausal women with early breast cancer. Front Oncol (2021) 11:690320. doi:10.3389/fonc.2021.690320
51. Keung, EZ, and Gershenwald, JE. The eighth edition American Joint Committee on Cancer (AJCC) melanoma staging system: implications for melanoma treatment and care. Expert Rev Anticancer Ther (2018) 18:775–84. doi:10.1080/14737140.2018.1489246
52. Hirsch, FR, Varella-Garcia, M, Bunn, PA, Di Maria, MV, Veve, R, Bremmes, RM, et al. Epidermal growth factor receptor in non-small-cell lung carcinomas: correlation between gene copy number and protein expression and impact on prognosis. J Clin Oncol Off J Am Soc Clin Oncol (2003) 21:3798–807. doi:10.1200/JCO.2003.11.069
53. McCarty, KS, Miller, LS, Cox, EB, and Konrath, J. Estrogen receptor analyses. Correlation of biochemical and immunohistochemical methods using monoclonal antireceptor antibodies. Arch Pathol Lab Med (1985) 109:716–21.
54. McCarty, KS, Szabo, E, Flowers, JL, Cox, EB, Leight, GS, Miller, L, et al. Use of a monoclonal anti-estrogen receptor antibody in the immunohistochemical evaluation of human tumors. Cancer Res (1986) 46:4244s–8s.
55. Ruengwanichayakun, P. Histochemical scoring assessment (H-score). Asian Arch Pathol (2021). Available from: https://www.asianarchpath.com/view/152 (Accessed July 20, 2024).
56. Dennis, MK, Burai, R, Ramesh, C, Petrie, WK, Alcon, SN, Nayak, TK, et al. In vivo effects of a GPR30 antagonist. Nat Chem Biol (2009) 5:421–7. doi:10.1038/nchembio.168
57. Wang, C, Prossnitz, ER, and Roy, SK. Expression of G protein-coupled receptor 30 in the hamster ovary: differential regulation by gonadotropins and steroid hormones. Endocrinology (2007) 148:4853–64. doi:10.1210/en.2007-0727
58. Peyton, C, and Thomas, P. Involvement of epidermal growth factor receptor signaling in estrogen inhibition of oocyte maturation mediated through the G protein-coupled estrogen receptor (Gper) in zebrafish (Danio rerio). Biol Reprod (2011) 85:42–50. doi:10.1095/biolreprod.110.088765
59. Gao, F, Ma, X, Ostmann, AB, and Das, SK. GPR30 activation opposes estrogen-dependent uterine growth via inhibition of stromal ERK1/2 and estrogen receptor alpha (ERα) phosphorylation signals. Endocrinology (2011) 152:1434–47. doi:10.1210/en.2010-1368
60. Patel, VH, Chen, J, Ramanjaneya, M, Karteris, E, Zachariades, E, Thomas, P, et al. G-protein coupled estrogen receptor 1 expression in rat and human heart: protective role during ischaemic stress. Int J Mol Med (2010) 26:193–9. doi:10.3892/ijmm_00000452
61. Uea, M, Salehi, SA, Windahl, S, Gomez, MF, Swärd, K, Daszkiewicz-Nilsson, J, et al. Deletion of the G protein-coupled receptor 30 impairs glucose tolerance, reduces bone growth, increases blood pressure, and eliminates estradiol-stimulated insulin release in female mice. Endocrinology (2009) 150:687–98. doi:10.1210/en.2008-0623
62. Haas, E, Bhattacharya, I, Brailoiu, E, Damjanović, M, Brailoiu, GC, Gao, X, et al. Regulatory role of G protein-coupled estrogen receptor for vascular function and obesity. Circ Res (2009) 104:288–91. doi:10.1161/CIRCRESAHA.108.190892
63. Wang, C, Dehghani, B, Magrisso, IJ, Rick, EA, Bonhomme, E, Cody, DB, et al. GPR30 contributes to estrogen-induced thymic atrophy. Mol Endocrinol Baltim Md (2008) 22:636–48. doi:10.1210/me.2007-0359
64. Brunsing, RL, and Prossnitz, ER. Induction of interleukin-10 in the T helper type 17 effector population by the G protein coupled estrogen receptor (GPER) agonist G-1. Immunology (2011) 134:93–106. doi:10.1111/j.1365-2567.2011.03471.x
65. Yates, MA, Li, Y, Chlebeck, PJ, and Offner, H. GPR30, but not estrogen receptor-alpha, is crucial in the treatment of experimental autoimmune encephalomyelitis by oral ethinyl estradiol. BMC Immunol (2010) 11:20. doi:10.1186/1471-2172-11-20
66. Jenei-Lanzl, Z, Straub, RH, Dienstknecht, T, Huber, M, Hager, M, Grässel, S, et al. Estradiol inhibits chondrogenic differentiation of mesenchymal stem cells via nonclassic signaling. Arthritis Rheum (2010) 62:1088–96. doi:10.1002/art.27328
67. Filardo, EJ, Graeber, CT, Quinn, JA, Resnick, MB, Giri, D, DeLellis, RA, et al. Distribution of GPR30, a seven membrane–spanning estrogen receptor, in primary breast cancer and its association with clinicopathologic determinants of tumor progression. Clin Cancer Res (2006) 12:6359–66. doi:10.1158/1078-0432.CCR-06-0860
68. Smith, HO, Arias-Pulido, H, Kuo, DY, Howard, T, Qualls, CR, Lee, SJ, et al. GPR30 predicts poor survival for ovarian cancer. Gynecol Oncol (2009) 114:465–71. doi:10.1016/j.ygyno.2009.05.015
69. Jung, J. Role of G Protein-Coupled estrogen receptor in cancer progression. Toxicol Res (2019) 35:209–14. doi:10.5487/TR.2019.35.3.209
70. Liu, H, Yan, Y, Wen, H, Jiang, X, Cao, X, Zhang, G, et al. A novel estrogen receptor GPER mediates proliferation induced by 17β-estradiol and selective GPER agonist G-1 in estrogen receptor α (ERα)-negative ovarian cancer cells. Cell Biol Int (2014) 38:631–8. doi:10.1002/cbin.10243
71. Yan, Y, Liu, H, Wen, H, Jiang, X, Cao, X, Zhang, G, et al. The novel estrogen receptor GPER regulates the migration and invasion of ovarian cancer cells. Mol Cell Biochem (2013) 378:1–7. doi:10.1007/s11010-013-1579-9
72. Smith, HO, Leslie, KK, Singh, M, Qualls, CR, Revankar, CM, Joste, NE, et al. GPR30: a novel indicator of poor survival for endometrial carcinoma. Am J Obstet Gynecol (2007) 196:386.e1–9. discussion 386.e9-11. doi:10.1016/j.ajog.2007.01.004
73. Petrie, WK, Dennis, MK, Hu, C, Dai, D, Arterburn, JB, Smith, HO, et al. G protein-coupled estrogen receptor-selective ligands modulate endometrial tumor growth. Obstet Gynecol Int (2013) 2013:472720. doi:10.1155/2013/472720
74. Avino, S, De Marco, P, Cirillo, F, Santolla, MF, De Francesco, EM, Perri, MG, et al. Stimulatory actions of IGF-I are mediated by IGF-IR cross-talk with GPER and DDR1 in mesothelioma and lung cancer cells. Oncotarget (2016) 7:52710–28. doi:10.18632/oncotarget.10348
75. Liu, C, Liao, Y, Fan, S, Fu, X, Xiong, J, Zhou, S, et al. G-Protein-Coupled estrogen receptor antagonist G15 decreases estrogen-induced development of non-small cell lung cancer. Oncol Res (2019) 27:283–92. doi:10.3727/096504017X15035795904677
76. Torres-López, L, Olivas-Aguirre, M, Villatoro-Gómez, K, and Dobrovinskaya, O. The G-protein-coupled estrogen receptor agonist G-1 inhibits proliferation and causes apoptosis in leukemia cell lines of T lineage. Front Cell Dev Biol (2022) 10:811479. doi:10.3389/fcell.2022.811479
77. Zhou, L, Yu, T, Yang, F, Han, J, Zuo, B, Huang, L, et al. G protein-coupled estrogen receptor agonist G-1 inhibits mantle cell lymphoma growth in preclinical models. Front Oncol (2021) 11:668617. doi:10.3389/fonc.2021.668617
78. Natale, CA, Li, J, Pitarresi, JR, Norgard, RJ, Dentchev, T, Capell, BC, et al. Pharmacologic activation of the G protein-coupled estrogen receptor inhibits pancreatic ductal adenocarcinoma. Cell Mol Gastroenterol Hepatol (2020) 10:868–80. doi:10.1016/j.jcmgh.2020.04.016
79. Hirtz, A, Lebourdais, N, Rech, F, Bailly, Y, Vaginay, A, Smaïl-Tabbone, M, et al. GPER agonist G-1 disrupts tubulin dynamics and potentiates temozolomide to impair glioblastoma cell proliferation. Cells (2021) 10:3438. doi:10.3390/cells10123438
80. Schüler-Toprak, S, Skrzypczak, M, Ignatov, T, Ortmann, O, and Treeck, O. G protein-coupled estrogen receptor 1 (GPER-1) and agonist G-1 inhibit growth of ovarian cancer cells by activation of anti-tumoral transcriptome responses: impact of GPER-1 mRNA on survival. J Cancer Res Clin Oncol (2020) 146:3175–88. doi:10.1007/s00432-020-03333-4
81. Lee, S-J, Kim, TW, Park, GL, Hwang, YS, Cho, HJ, Kim, J-T, et al. G protein-coupled estrogen receptor-1 agonist induces chemotherapeutic effect via ER stress signaling in gastric cancer. BMB Rep (2019) 52:647–52. doi:10.5483/BMBRep.2019.52.11.007
82. Ambrosini, G, Natale, CA, Musi, E, Garyantes, T, and Schwartz, GK. The GPER agonist LNS8801 induces mitotic arrest and apoptosis in uveal melanoma cells. Cancer Res Commun (2023) 3:540–7. doi:10.1158/2767-9764.CRC-22-0399
83. Linnaeus Therapeutics, Inc. Study assessing MTD, safety, tolerability, PK and anti-tumor effects of LNS8801alone and with pembrolizumab. clinicaltrials.gov (2022). Available from: https://clinicaltrials.gov/study/NCT04130516?intr=LNS8801&rank=1 (Accessed August 18, 2022).
84. Liu, C-C, Lin, J-H, Hsu, T-W, Hsu, JW, Chang, JW, Su, K, et al. Collagen XVII/laminin-5 activates epithelial-to-mesenchymal transition and is associated with poor prognosis in lung cancer. Oncotarget (2018) 9:1656–72. doi:10.18632/oncotarget.11208
85. Yamada, T, Endo, R, Tsukagoshi, K, Fujita, S, Honda, K, Kinoshita, M, et al. Aberrant expression of a hemidesmosomal protein, bullous pemphigoid antigen 2, in human squamous cell carcinoma. Lab Investig J Tech Methods Pathol (1996) 75:589–600.
86. Thangavelu, PU, Krenács, T, Dray, E, and Duijf, PHG. In epithelial cancers, aberrant COL17A1 promoter methylation predicts its misexpression and increased invasion. Clin Epigenetics (2016) 8:120. doi:10.1186/s13148-016-0290-6
87. Tamás, L, Szentkúti, G, Eros, M, Dános, K, Brauswetter, D, Szende, B, et al. Differential biomarker expression in head and neck cancer correlates with anatomical localization. Pathol Oncol Res POR (2011) 17:721–7. doi:10.1007/s12253-011-9376-9
88. Parikka, M, Kainulainen, T, Tasanen, K, Väänänen, A, Bruckner-Tuderman, L, and Salo, T. Alterations of collagen XVII expression during transformation of oral epithelium to dysplasia and carcinoma. J Histochem Cytochem Off J Histochem Soc (2003) 51:921–9. doi:10.1177/002215540305100707
89. Parikka, M, Nissinen, L, Kainulainen, T, Bruckner-Tuderman, L, Salo, T, Heino, J, et al. Collagen XVII promotes integrin-mediated squamous cell carcinoma transmigration--a novel role for alphaIIb integrin and tirofiban. Exp Cell Res (2006) 312:1431–8. doi:10.1016/j.yexcr.2006.01.015
90. Hwang, B-J, Zhang, Y, Brozowski, JM, Liu, Z, Burette, S, Lough, K, et al. The dysfunction of BP180/collagen XVII in keratinocytes promotes melanoma progression. Oncogene (2019) 38:7491–503. doi:10.1038/s41388-019-0961-9
91. Tong, D, Tanaka, M, Eguchi, H, Okazaki, Y, Muramatsu, M, and Arai, T. COL17A1 germline variant p.Ser1029Ala and mucosal malignant melanoma: an autopsy study. Mol Clin Oncol (2022) 16:32. doi:10.3892/mco.2021.2465
92. Lothong, M, Sakares, W, Rojsitthisak, P, Tanikawa, C, Matsuda, K, and Yodsurang, V. Collagen XVII inhibits breast cancer cell proliferation and growth through deactivation of the AKT/mTOR signaling pathway. PLoS One (2021) 16:e0255179. doi:10.1371/journal.pone.0255179
93. Fujiwara, S, Terai, Y, Kawaguchi, H, Takai, M, Yoo, S, Tanaka, Y, et al. GPR30 regulates the EGFR-Akt cascade and predicts lower survival in patients with ovarian cancer. J Ovarian Res (2012) 5:35. doi:10.1186/1757-2215-5-35
94. Chen, J, Zhao, R, Wang, Y, Xiao, H, Lin, W, Diao, M, et al. G protein-coupled estrogen receptor activates PI3K/AKT/mTOR signaling to suppress ferroptosis via SREBP1/SCD1-mediated lipogenesis. Mol Med (2024) 30:28. doi:10.1186/s10020-023-00763-x
95. Wang, Y, Peng, Z, Meng, R, Tao, T, Wang, Q, Zhao, C, et al. NHERF1 inhibits proliferation of triple-negative breast cancer cells by suppressing GPER signaling. Oncol Rep (2017) 38:221–8. doi:10.3892/or.2017.5649
Keywords: estrogen receptor, collagen xvii, prognostic factors, immunohistochemistry, prognosis
Citation: Çakır U, Balogh P, Ferenczik A, Brodszky V, Krenács T, Kárpáti S, Sárdy M, Holló P and Fábián M (2024) G protein-coupled estrogen receptor 1 and collagen XVII endodomain expression in human cutaneous melanomas: can they serve as prognostic factors?. Pathol. Oncol. Res. 30:1611809. doi: 10.3389/pore.2024.1611809
Received: 28 April 2024; Accepted: 14 August 2024;
Published: 26 August 2024.
Edited by:
Anna Sebestyén, Semmelweis University, HungaryCopyright © 2024 Çakır, Balogh, Ferenczik, Brodszky, Krenács, Kárpáti, Sárdy, Holló and Fábián. This is an open-access article distributed under the terms of the Creative Commons Attribution License (CC BY). The use, distribution or reproduction in other forums is permitted, provided the original author(s) and the copyright owner(s) are credited and that the original publication in this journal is cited, in accordance with accepted academic practice. No use, distribution or reproduction is permitted which does not comply with these terms.
*Correspondence: Uğur Çakır, cakir.ugur@semmelweis.hu