- 1Department of Experimental Pharmacology, National Institute of Oncology, Budapest, Hungary
- 22nd Department of Pediatrics, Semmelweis University, Budapest, Hungary
- 3Department of Otolaryngology and Head and Neck Surgery, Semmelweis University, Budapest, Hungary
- 42nd Institute of Pathology, Semmelweis University, Budapest, Hungary
- 5Hungarian Cancer Registry, National Institute of Oncology, Budapest, Hungary
- 6Department of Thoracic Surgery, Medical University of Vienna, Vienna, Austria
- 7Department of Tumor Biology, National Korányi Institute of Pulmonology, Budapest, Hungary
- 8Vichem Chemie Ltd., Budapest, Hungary
Background: Anti-EGFR antibody therapy is still one of the clinical choices in head and neck squamous cell carcinoma (HNSCC) patients, but the emergence of cetuximab resistance questioned its effectiveness and reduced its applicability. Although several possible reasons of resistance against the antibody treatment and alternative therapeutic proposals have been described (EGFR alterations, activation of other signaling pathways), there is no method to predict the effectiveness of anti-EGFR antibody treatments and to suggest novel therapeutics. Our study investigated the effect of EGFR R521K alteration on efficiency of cetuximab therapy of HNSCC cell lines and tried to find alternative therapeutic approaches against the resistant cells.
Methods: After genetic characterization of HNSCC cells, we chose one wild type and one R521K+ cell line for in vitro proliferation and apoptosis tests, and in vivo animal models using different therapeutic agents.
Results: Although the cetuximab treatment affected EGFR signalization in both cells, it did not alter in vitro cell proliferation or apoptosis. In vivo cetuximab therapy was also ineffective on R521K harboring tumor xenografts, while blocked the tumor growth of EGFR-wild type xenografts. Interestingly, the cetuximab-resistant R521K tumors were successfully treated with c-MET tyrosine kinase inhibitor SU11274.
Conclusion: Our results suggest that HNSCC cell line expressing the R521K mutant form of EGFR does not respond well to cetuximab treatment in vitro or in vivo, but hopefully might be targeted by c-MET tyrosine kinase inhibitor treatment.
Introduction
Over 600,000 head and neck squamous cell carcinoma (HNSCC) patients are diagnosed annually worldwide [1]. In Hungary, they represent the third most abundant type of malignant tumors among men [2]. Patients with early (I-II) stage localized HNSCC undergo radiation or surgery as monotherapy, while patients with advanced tumors (stage III-IV) are treated by combination therapy [3]. The 5-years survival of these patients still remains less than 50% [4,5] therefore finding new therapeutic regimes is an urgent need for clinical practice.
As in many epithelial tumor types, EGFR protein is overexpressed in HNSCC, and increased EGFR gene copy number is frequently described in this region as well [6–9]. Due to its molecular characteristics EGF receptor seemed to be a promising target in the treatment of HNSCC.
At present, there are two different ways to inhibit signaling pathway via EGF receptor. First, the use of anti-EGFR monoclonal antibodies (for example cetuximab, C225, Erbitux®) which bind to the extracellular ligand binding domain of the receptor. Second, using low molecular weight tyrosine kinase inhibitors (for example gefitinib, ZD 1839, Iressa®, or erlotinib, OSI 774, Tarceva®) targeting the intracellular tyrosine kinase domain of the receptor.
Cetuximab has been extensively studied in preclinical models and clinical experiments as well. As former studies have shown [10,11], nowadays, cetuximab is indicated to be used in two stages of the treatment of HNSCC: it was approved by FDA for the treatment of locally or regionally advanced HNSCC in part of chemoradiotherapy, or as monotherapy for patients with relapsed or metastatic tumors, whose platinum-based treatment has formerly failed [12].
The antitumor activity of low molecular weight tyrosine kinase inhibitors (TKIs) has been studied thoroughly in many EGFR overexpressing solid tumors: non-small cell lung cancer (NSCLC), pancreatic cancer, glioblastoma and head and neck cancer. Erlotinib treatment has a significant effect on survival in patients with NSCLC, however, it showed only a modest activity in HNSCC patients [13]. Introducing gefitinib in the therapy of head and neck tumors seemed to be promising based on the molecular characteristics of HNSCCs, nevertheless, in many clinical trials survival benefit and response rate were very low [14,15].
Despite the fact that, based on experimental data, EGFR seemed to be one of the most promising molecular targets in HNSCC, it did not always meet the expectations in clinical practice. Previous results showed that only 10–20% of cancer patients have major clinical responses to EGFR inhibition, either because they barely respond primarily or they acquire resistance during anti-EGFR treatment [16]. Taken together, these data showed that the usage of EGFR inhibition does not necessarily lead to a considerably improved survival rate [17].
The EGFR genotype and phenotype have been reported to have dramatic effect on anti-EGFR therapy efficacy. The classical mutations already used in multiple cancer diagnostics and therapy selection are affecting the intracellular tyrosine kinase domain (exons 19–22). Their role is clear in overcoming TKI therapy efficacy. However, much less is known about the extracellular variations of the receptor, which might confer resistance or hypersensitivity to antibody treatment. In HNSCC, EGFRvIII variant (suffering deletion of exons 2–7), and single nucleotide polymorphism R521K are reported. However, according to our ongoing screening on clinical samples of HNSCC patients, EGFRvIII was not found in more than 1 percent of the patients, therefore was ruled out as possible explanation of different patient response. Importantly, the missense mutation R521K, was quite abundant in our clinical cohort, and, knowing that its position is in the neighborhood of the cetuximab-binding site of the receptor [18], we focused on its possible correlations with in vitro, in vivo and clinical response to cetuximab-based therapy.
A potent mechanism that may cause resistance to anti-EGFR therapy is activation of alternative receptor tyrosine kinases and signaling pathways (PI3K/AKT, c-Met, Src) [19,20]. The usage of the specific inhibitors of these other kinases in monotherapy or in combination with EGFR inhibition may have increase clinical efficacy in anti-EGFR therapy resistant HNSCC tumors [16,21].
Hepatocyte growth factor (HGF) and its receptor, c-MET are major regulators of cell proliferation, survival, migration and angiogenesis. In tumor cells they contribute to proliferation, apoptosis inhibition, invasion, as well as adhesion. Eventually the HGF-c-MET system confers tumor progression and metastasis. c-MET protein overexpression has been reported in various human epithelial cancer types including HNSCC. Moreover, c-MET has been found in increased amount in lymph node metastases of HNSCC compared with primary tumors [22,23]. This suggests that c-MET has a pivotal role in the development of the metastatic potential of HNSCCs [22].
In the current experimental study, we have investigated the effect of different EGFR inhibitors (cetuximab and erlotinib), the specific c-MET TK inhibitor SU11274 and the RAS protein specific inhibitor zoledronic acid on proliferation and apoptosis of human squamous cell carcinoma cells in vitro as well as on the growth and metastasis of HNSCC xenografts in vivo, alone and in combination as well.
Methods
Cell Lines and Culture Conditions
The PE/CA-PJ15 (oral SCC from a 45-year-old man, ECACC No: 96121230) and PE/CA-PJ41 (oral SCC from a 67-year-old woman, ECACC No: 98020207) human head and neck squamous carcinoma cell lines were obtained from ATCC. Cells were grown in Iscove’s Modified Eagle Medium (IMEM, Sigma-Aldrich, St. Louis, MO), with the addition of 10% fetal bovine serum (Sigma) and 1% penicillin-streptomycin (Sigma) in a 37°C incubator, using 5% CO2 concentration.
Identification of EGFR Extracellular Domain Genetic Alterations and Quantitative Measurement of mRNA Expression of EGFR in HNSCC Cell Lines
Total RNA was isolated from the in vitro growing tumor cell cultures using TRIzol® Reagent (Invitrogen, Carlsbad, CA, United Status) and Direct-zol RNA miniprep kit (Zymo research, Irvine, CA), according to the manufacturer’s instructions. Possible DNA contamination was eliminated using Dnase I treatment. For reverse transcription, 1 μl of 10 mM dNTP mix (Finnzyme, Espoo, Finland) and 1 μl of Random primer-oligo dT was added and transcribed 2 μg of the purified genomic RNA. After incubation of the mixture at 70°C for 10 min, 2 μl of 10× M-MLV Reverse transcriptase Buffer (Sigma), 1 μl of M-MLV Reverse transcriptase (200 units/μl, Sigma) enzyme, 0.5 μl RNase Inhibitor (40 units/μl, Promega, Madison, WI 53711, United States) and 6.5 μl nuclease-free water were added. The reactions were incubated at 37°C for 50 min and 85°C for 10 min. Successful reverse transcription was proved by PCR reactions with β-actin primers as a housekeeping gene probe, followed by agarose gel electrophoresis. DNA contamination of the template RNA and nuclease-free water was also controlled every time.
For detecting transcribed EGFR-ECD, serial PCR reactions were carried out with seven different nested primer pairs designed by Array Designer Oligo and cDNA Microarray Design Software (Premier Biosoft International). The primers were optimized to target reference sequence NM_005228.3. The PCR reactions contained 11.5 μl AmpliTaq® Gold 360 MasterMix (Applied Biosystems), 2.5–2.5 μl of the primers (see primers in Table 1), 2 μl of the cDNA template, and 6.5 μl nuclease free water. The reaction program included: 95°C for 10 min, then 38 cycles of 95°C for 1 min, 55°C for 1 min, 72°C for 2 min. Final extension was at 72°C for 5 min. PCR products were separated on a 2% agarose gel and captured with the MULTI GENIUS Bio Imaging System (Syngene, Frederick, MD), recording visual signal of ethidium bromide labeling applied. Transcribed fragments were identified according to the size of the separated PCR products. The proper band was excised and DNA was isolated using the QIAquick Gel Extraction Kit (Qiagen, Valencia, CA, United States). The purified PCR fragments of EGFR-ECD were analyzed by direct sequencing in both directions. BigDye® Terminator v1.1 Cycle Sequencing Kit (Applied Biosystems™–by Life Technologies™) was used for the sequencing, according to the manufacturer’s protocol (the primers were identical to those used at PCR amplification). We purified the sequencing reaction products using BigDye® XTerminator™ Purification Kit (Applied Biosystems™–by Life Technologies™). PCR products were analyzed by a 4-capillary automated sequencer (Applied Biosystems 3130 Genetic Analyzer, Applied Biosystems).
For the detection of the expressed EGFRvIII isoform, a variant-specific PCR reaction (primers shown in Table 1) was used. Besides HNSCC cell lines, we used U87vIII glioblastoma cell line (Sigma)—transduced with EGFR vIII isoform—sample as a positive control. The reactions were processed in 20 μl volume containing 10 μl 2 × GoTaq Green PCR mix, 1 μl forward and reverse primer, 500 ng cDNA template, and nuclease-free water was added to reach the final volume. The PCR protocol included activation phase at 94°C for 4 min, 35 polymerization cycles (94°C for 30 s—60°C for 30 s—72°C for 1 min), and a final elongation phase at 72°C for 10 min. The PCR products were then separated in 1% agarose gel horizontal electrophoresis system using EcoSafe (Pacific Image Electronics, Taiwan) gel stain at a dilution of 1:20,000 in order to visualize DNA for photographing.
In parallel, we measured the quantity of wild type version of EGFR (primer is3/ia3) by real-time PCR reaction measurements. Reaction mixtures contained 12.5 μl ABsolute™ QPCR SYBR® Green Mix (Thermo Fisher Scientific Carlsbad, CA), 0.5–0.5 μl of each primer (reaching primer concentration of 200 nM) and 11.5 μl of the cDNA template. The cycling conditions (LightCycler 480 II, Roche Diagnostics, Basel, Switzerland) included 3 minutes of DNA polymerase activation at 95°C, and 40 cycles of the following steps: 95°C for 30 s, 55°C for 30 s, 72°C for 60 s. Starting quantities were defined on the basis of standard dilution series (1×-625×). cDNA of PC3 human prostate carcinoma cell line was used as control template.
The cell lines were also examined for KRAS, NRAS, EGFR tyrosine kinase domain genotypes and HPV (see primers in Supplementary Table S1).
Immunocytochemistry of HNSCC Cells
To detect EGFR, c-MET or the activated receptor (phospho-EGFR, and phospho-c-MET) proteins, HNSCC cells were fixed in 4% paraformaldehyde for 10 minutes, permeabilized using 0.1% Triton X-100 (Sigma) PBS for 1 min. After washing, we blocked non-specific reactions with 1% bovine serum albumin (BSA; Sigma) for 30 min at room temperature. Samples were then incubated with the following primary antibodies: EGF Receptor (D38B1) XP® Rabbit mAb #4267, Phospho-EGF Receptor (Tyr1068) (D7A5) XP® Rabbit mAb #3777, Met (D1C2) XP® Rabbit mAb #8198, Phospho-Met (Tyr1234/1235) (D26) XP® Rabbit mAb #3077. All primary antibodies were obtained from Cell Signaling Technology (Danvers, MA). We incubated the samples at 37°C for one hour, followed by washing in PBS for 3 × 10 min, and incubated with Alexa-488 conjugated goat anti-rabbit IgG (H + L) antibody (Thermo Fisher Scientific) for 45 min at 37°C (dilution 1:100). Negative controls were prepared by replacing the primary antibody with isotype-matched non-immune IgG (Sigma). Cell nuclei were stained with 1 μg/ml Hoechst 33,342 (Sigma) for 2 min at room temperature. Slides were covered with Prolong Gold antifade reagent (Thermo Fisher Scientific), and cells were photographed using fluorescent microscopy (Leica DM IL LED Microscope with mercury lamp and Leica DFC345 FX camera). All images were produced with the same exposure setup for the same channel.
EGFR, RAS and c-MET Inhibitors
EGFR-specific humanized monoclonal antibody (cetuximab, IMC-225, Erbitux®; Merck) was used at concentrations of 10 and 100 μg/ml in RPMI for in vitro, and at 400 mg/m2 loading dose followed by 250 mg/m2 sustaining dose in physiological salt solution weekly for in vivo studies.
EGFR specific small molecule TKI erlotinib (OSI 774, Tarceva®; Roche) was used at concentrations of 5 and 25 μM for in vitro studies. In animal experiments, SCID mice were treated at human equivalent dose daily with erlotinib at 2 mg/kg.
In our setup, we also used the RAS inhibitor zoledronic acid (Zometa; Novartis Europharm), at concentrations of 10 and 50 μM in cell proliferation assays, and 50 μg/kg for in vivo experiments.
Cetuximab, erlotinib, and zoledronic acid were used in aqueous stock solutions, and diluted in cell culture medium for in vitro assays, or physiological saline with in vivo experiments.
The c-MET-specific inhibitor [24] SU11274 [(3Z)-N-(3-chlorophenyl)-3-({3,5-dimethyl-4-[(4-methylpiperazin-1-yl)carbonyl]-1H-pyrrol-2-yl}methylene)-N-methyl-2-oxo-2,3-dihydro-1H-indole-5-sulfonamid] (Pfizer Inc, San Diego, CA; synthesized by Vichem Chemie Ltd, Budapest, Hungary) was dissolved in DMSO (Sigma) to form 5 mM solution, and was used in 1 and 5 μM concentrations for in vitro studies and 0.5 mg/kg dose was applied for in vivo assays. Appropriate concentrations of DMSO solutions were used as control both in vitro and in vivo.
Cell Proliferation Assay
HNSCC cells were plated at 2 × 103 viable cells/well on 96-well plates (Greiner, Frickenhausen, Germany) and after attachment to the surface, treated with the EGFR, RAS and c-MET inhibitors at the concentrations described above for 48 h. All treatments were performed in serum-containing or serum-free medium, in a total volume of 200 μl. After the incubation, we added 20 μl of 5 mg/ml thiazolyl blue tetrazolium bromide (MTT, Sigma) to the wells, and incubated for another 4 h at 37°C, then removed the solution, and dissolved the cell culture in 100 μl 1:1 mixture of DMSO and ethyl-alcohol (Sigma). Absorbance was recorded at 570 nm using a microplate Reader (BioRad, Hercules, CA).
Flow Cytometric Measurement for Apoptosis
PJ15 and PJ41 cell suspensions (1.5 × 105 viable cells/well) were put in 6-well plates, and treated for 48 h with different concentrations of inhibitors: cetuximab (10 or 100 μg/ml), erlotinib (5 or 25 μM), zoledronic acid (10 or 50 μM) and SU11274 (1 or 5 μM). Then, they were detached with 0.02% EDTA solution (Sigma), washed twice with PBS. After centrifugation for 5 min by 1000 rpm, cells were fixed in 1 ml 70% ethanol at –20°C for 30 min and washed in PBS. We added 2 ml Staining Buffer containing 12 μl propidium iodide and 6 μl RNase (Partec, Munster, Germany) to each sample. After 4 h of incubation we quantified the total DNA content in the cells by flow cytometry (Cy-Flow SL-Green, Partec). Apoptotic cells were determined as the amount of cells in the sub-G1 state of propidium iodide (PI) staining. Samples were analyzed by the FlowMax software Sysmex (Partec, Görlitz, Germany).
Flow Cytometric Measurement of c-MET and EGFR Protein Expression and Activation
Cultured cells were detached using 0.02% EDTA solution, washed two times with serum-free medium, and then fixed/permeabilized in 1% ice-cold methanol for 15 min. Nonspecific binding sites were blocked with 3% BSA for 15 min. After that, cells were labeled with the following antibodies: polyclonal rabbit antibody against the intracellular domain of EGFR (1:10 dilution in PBS, PU335-UP, BioGenex, San Remon, CA); phospho-specific polyclonal rabbit antibody binding to the phospho-tyrosine autophosphorylation site 1068 on the tyrosine kinase domain (1:100 dilution in PBS, 44–788, Biosource International Inc., Camarillo, CA); polyclonal rabbit antibody against the intracellular domain of c-MET (1:50 dilution in PBS, C-12, Santa Cruz Biotechnology, CA) and rabbit anti-c-MET [pYpYpY1230/1234/1235] phosphospecific antibody (1:20 dilution in PBS, Biosource, Nivelles, Belgium) for 45 min at 37°C. After washing thrice for 5 min (PBS), FITC-conjugated polyclonal goat anti-mouse antibody (DakoCytomation, Glostrup, Denmark) was used as secondary antibody. Fluorescence values were detected by FACSCalibur flow cytometer (Becton Dickinson, Sunnyvale, CA). Positive events were counted from at least a total of 104 cells. Negative controls were prepared using isotype control IgG (Sigma).
Western Blot Analysis
One million adherent cells cultured and drug treated in T25 cell culture flasks were lysed with MLB (No. 20–168, Merck KGaA, Darmstadt, Germany) cell lysis solution containing Halt protease inhibitor cocktail (Fisher Scientific) and 0.1 mg/ml PMSF to inhibit protein and phospho-protein degradation. Following SDS-PAGE and blotting, protein levels were detected using the following primary antibodies: rabbit polyclonal pERK1/2 and ERK1/2 (#9101 and #9102, respectively, Cell Signaling Technology), at a dilution of 1:1,000, phosphospecific polyclonal rabbit antibody against phospho-tyrosine 1,068 (Biosource 44–788) at a dilution of 1:500, and mouse IgG1 antibody against the extracellular domain of EGFR (Clone E30, M7239, Dako). We detected antibody signals using appropriate HRP-conjugated goat secondary antibodies (Jackson ImmunoResearch, West Grove, PA, United States). Immunoblots were revealed by ECL Reagent (GE Healthcare, Dassel, Germany).
Anti-EGFR Therapy Effects on Subcutaneously Growing Tumor Xenografts
SCID (CB17/Icr-Prkdcscid) mice were originated from our inbred and housed SPF (specific pathogen-free) mouse colony. Not more than 10 animals were caged together. Previously cultured and trypsinized PE/CA-PJ15, and PE/CA-PJ41 cell suspensions washed with serum-free medium was inoculated subcutaneously into the back of 8–10-week old treatment-naïve male SCID mice, at a quantity of 1.6 × 106 cells/animal, 7 animals in each groups. Twenty-four days following subcutaneous injection of the PJ15 and thirty-six days following the injection of the PJ41 tumor cells, measurable tumors were developed, the animals were assigned to treatment groups in order to have the same tumor size distribution at the beginning of the treatment. To record the effects of targeted therapies on tumor growth, animals were treated intraperitoneally (i.p.) with the following inhibitors for three or four weeks (PJ15 and PJ41, respectively): erlotinib (2 mg/kg, daily), cetuximab (400 + 250 mg/m2, weekly), zoledronic acid (50 μg/kg, weekly), erlotinib + cetuximab, erlotinib + zoledronic acid, cetuximab + zoledronic acid, erlotinib + cetuximab + zoledronic acid and physiological saline solution as negative control.
The volumes of the subcutaneously growing tumors were determined by the measurement of two diameters by caliper twice weekly from the first treatment. Tumor volumes were estimated using the formula [length × width2 × π/6]. The treated groups were compared to untreated group; the relative effects of the treatments were analyzed using percentages of the control tumor growth.
Murine Liver Colonization Assay for Human Tumor Xenografts
Previously cultured human HNSCC PE/CA-PJ15 and PJ41 cells were trypsinized, washed twice with serum-free medium, and suspension containing 5 × 105 cells was inoculated into the spleen of anesthetized 8–10-week old treatment-naïve male SCID mice (8 animals in each group). Twenty-eight days after tumor cell injection, intraperitoneal administration of 0.5 mg/kg SU11274 or solvent control occurred daily for 3 weeks. At the endof the experiment, tumors were excised, tumor mass was measured, and macroscopic liver colonies were counted using a stereomicroscope. The effect of SU11274-treatment was determined based on tumor weight or metastasis number as a percentage of those of the control group. Tumor cells were labeled with anti-cytokeratin antibody (AE1/AE3, M3515, Dako) according to manufacturer’s recommendations using AEC as chromogen.
Animal Housing and Treatment Conditions
All animal housing and breeding processes (permission No: PEI/001/1738-3/2015) and animal experiments (permission No: PEI/001/2574–6/2015) were conducted following standards and procedures approved by the Animal Care and Use Committee of the National Institute of Oncology, Budapest. Group sizes were reduced to minimize animal use, while representing well the individual variances inside the group. All (112/112) animals inoculated subcutaneously were included in the analysis. In the liver metastasis model, we excluded five animals where no visible sign of primary or metastatic tumor burden occurred, signaling inefficient intrasplenic injection. In total, 27/32 animals were included in the metastasis analysis. All inoculations and treatments were performed under a sterile laminar biosafety cabinet (between 1:00 PM and 3:00 PM each treatment day). Tumor cell inoculation was performed always on animals anesthetized by the intraperitoneal injection of the mixture of zolazepam (20 mg/kg), xylazine (12.5 mg/kg), butorphanol (3 mg/kg), and tiletamine (20 mg/kg). Assessment of proper anesthetization was controlled by pedal reflex and monitoring respiratory rate. All animals were euthanized using anesthetization by isoflurane inhalation followed by cervical dislocation. Our animal house follows FELASA health status control recommendation to monitor whether all our strains are free from pathogens or other diseases. All animals recruited to the experimental group weighed between 22 and 25 g at the beginning of the experiment, and no more than 10% body weight loss was observed through the experiments.
Statistics
To determine statistical differences between two groups Student’s t-test was used. For statistical analysis of differences among more than two groups ANOVA was used with the post hoc Scheffé-test, where parametric methods were available. For the animal experiments we used the non-parametric Mann-Whitney U-test to evaluate treatment effects. Statistical significance was determined and marked as following: *: p < 0.05; **: p < 0.01; ***: p < 0.001. Statistical analysis was realized using Statistica 12.0 software (StatSoft, Tulsa, OK).
Results
Effect of the EGFR Polymorphism R521K on the In Vitro Sensitivity of HNSCC Cell Lines to the EGFR Specific Therapeutic Antibody Cetuximab, and Small-Molecule Inhibitors of EGFR, RAS, and c-MET
To investigate the possible effects of EGFR genetic alterations on the sensitivity of HNSCC cell lines to the EGFR-directed antibody cetuximab, the EGFR specific tyrosine kinase inhibitor (TKI) erlotinib, the RAS inhibitor zoledronic acid, and the c-MET inhibitor SU11274, we chose two cell lines, the PE/CA-PJ15 harboring one R521K mutant allele of EGFR (heterozygous) and the PE/CA-PJ41 cell line expressing only wild type EGFR (homozygous wild type, Figure 1A). Molecular biological analysis of the extracellular domain also revealed two other synonym SNPs in the EGFR gene (Figure 1A). The two cell lines were found to be negative for the EGFRvIII isoform (deletion of 2-7 exons, results shown on Figure 1B) and for the mutations of the TK domain (regions exon 19 and exon 21), and did not contain any alterations in the hot point regions of KRAS and NRAS, either (see Supplementary Table S2).
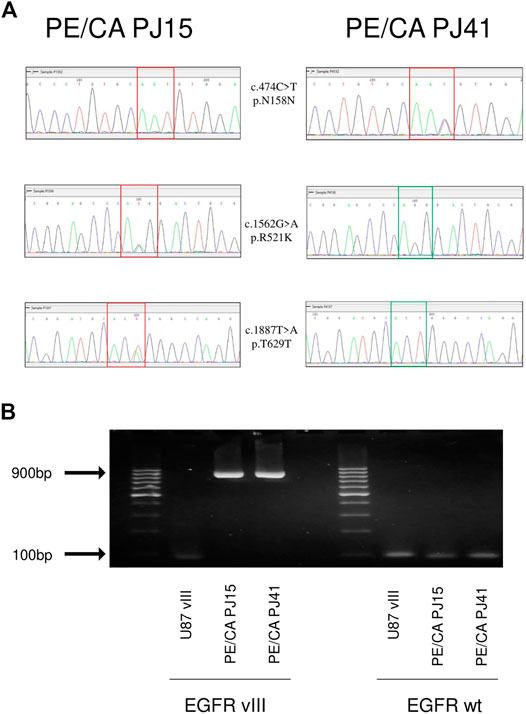
FIGURE 1. Genetic alterations of EGFR extracellular domain in PE/CA-PJ15 and PJ41 HNSCC cell lines (A) Different SNP pattern in the case of two PE/CA cell lines: PE/CA-PJ15 c720 C > T Asn158Asn homozygous SNP (upper row, left), PE/CA-PJ41 c720 C > T Asn158Asn heterozygous SNP (upper row, right), PE/CA-PJ15 c1808 G > A, Arg521Lys (middle row left); PE/CA-PJ15 c2133 T > A Thr629Thr (lower row, left). (B) investigation of EGFR vIII and EGFR wild type isoforms after mRNA isolation, reverse transcription, PCR and agarose gel electrophoresis. The left side shows that U87 vIII, an EGFR vIII overexpressing glioblastoma cell line expresses the vIII isoform of the receptor, while the two HNSCC cell lines express only the untruncated wild type isoform, showing the amplification of a large region of 896 base pairs. The right side of the image shows that wild type EGFR is present in all of the samples.
To evaluate the sensitivity of HNSCC cell lines, first we investigated the in vitro effects of EGFR, RAS and c-MET inhibitors on cancer cell proliferation. While the EGFR specific TK inhibitor erlotinib and the RAS inhibitor zoledronic acid significantly decreased in vitro proliferation of the two HNSCC cell lines both in serum-containing and serum-free media (Figures 2A,B), interestingly, the therapeutic anti-EGFR antibody cetuximab showed no effect on cancer cell proliferation either in the case of the PE/CA-PJ15 cell line harboring the R521K EGFR polymorphism or in the homozygous wild type PE/CA-PJ41 cells. Moreover, we found that the specific c-MET inhibitor SU11274 at higher concentration efficiently decreased cancer cell proliferation in both PE/CA-PJ41 and PJ15 cells. However, the sensitivity of the two cell lines against the specific small-molecule inhibitors was different as shown in Figures 2A,B. PE/CA-PJ15 cell line had higher sensitivity in all cases, especially when cells were treated with the c-MET inhibitor SU11274. To further investigate the similar cetuximab resistant behavior of the two cell lines, we also measured the combined effects of the inhibitors. We observed that the toxicity of cetuximab was not augmented by the presence of the RAS inhibitor zoledronic acid even at high antibody concentration (100 μg/ml) verifying the in vitro cetuximab resistant phenotype of PE/CA-PJ15 and PJ41 cells. Contrarily, the combination of erlotinib and zoledronic acid showed clear additive cytotoxic effects in PE/CA-PJ41 cells. Next, we monitored drug/antibody induced apoptosis in HNSCC cell lines on the basis of the decreased DNA content of apoptotic cells (sub-G1 population) by flow cytometric analysis. Consistent with our previous results, cetuximab treatment did not induce apoptosis in any of the two cell lines confirming in vitro ineffectiveness of cetuximab (Figure 2C). Contrarily, small-molecule inhibitors significantly increased apoptotic cell number in both cell lines. Moreover, the c-MET inhibitor SU11274 provoked dramatic cell death in PE/CA-PJ15 cells, while in PE/CA-PJ41 cells the apoptotic cell number did not increase above the baseline (untreated control).
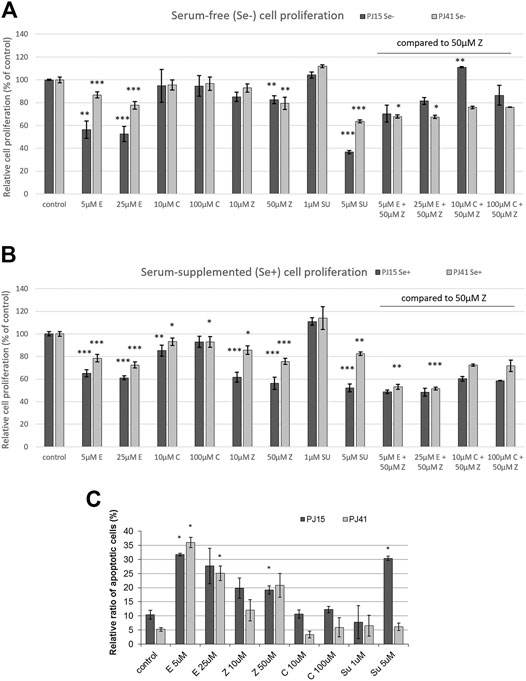
FIGURE 2. Effects of investigated inhibitors on the in vitro proliferation and apoptosis of HNSCC cell lines. (A,B) While therapeutic antibody cetuximab had no effect on in vitro cell proliferation in any of the two cell lines, small molecule EGFR- and c-MET-specific TKIs and RAS inhibitors were found to be more effective against PJ15 cells compared to PJ41. The combined therapy of HNSCC cells with anti-EGFR compounds shows that cetuximab did not affect cell proliferation, not even in combination, but TKIs and RAS inhibitor have pronounced antiproliferative effect on the two cell lines, which was much stronger on PJ15 cells than on PJ41 cells. Graphs show mean ± SD of four parallel samples, for single drug treatments, and two parallels of combination treatments, quantified as % of control (untreated cells). All values were measured in triplicates. (C) Flow cytometric determination of apoptotic nuclei (subG1 fraction). Erlotinib and zoledronic acid significantly increased the apoptosis in both HNSCC cell lines. However, c-MET-specific inhibitor SU11274 induced apoptosis in the PJ15 cells only. Cetuximab had no effect similarly to results of the proliferation assay. Data are means ± SD of three parallel samples “C”: cetuximab; “E”: erlotinib, “Z”: zoledronic acid; “SU”: SU11274.
In Vivo Cetuximab Resistance of PE/CA-PJ15 Cells Harboring the R521K EGFR Polymorphism
To characterize the in vivo sensitivity of HNSCC cells against the specific inhibitors, SCID mice bearing PE/CA-PJ15-, and PE/CA-PJ41-derived xenografts were treated with the inhibitors intraperitoneally in human equivalent doses. Interestingly, in contrast to our in vitro results, the two HNSCC cell lines showed markedly different sensitivity to cetuximab. In the case of PE/CA-PJ15 cells harboring the R521K EGFR mutation, cetuximab did not affect tumor growth. However, the treatment with erlotinib or zoledronic acid significantly decreased PJ15 tumor volumes (Figure 3A). Moreover, combined treatments acted additively in all therapeutic regimens confirming the ineffectiveness of cetuximab and revealing the most efficient drug combination of erlotinib and the RAS inhibitor zoledronic acid that dramatically eliminated cetuximab resistant tumors (Figure 3A). Contrarily, cetuximab showed profound inhibitory effect on the growth rate of PE/CA-PJ41-derived xenografts expressing only wild type EGFR, and tumors were completely eradicated upon treatment with all combined therapeutic regimes containing cetuximab (Figure 3B). Interestingly, in the case of PE/CA-PJ41-derived tumors, the combination of erlotinib and zoledronic acid did not enhance treatment efficiency but showed the same inhibitory effect as the drugs alone.
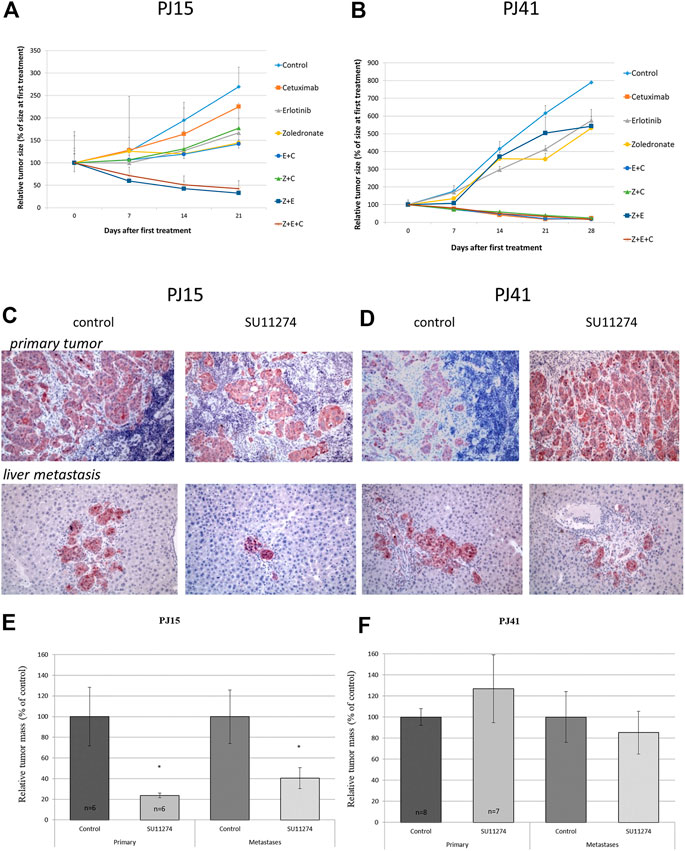
FIGURE 3. In vivo effect of EGFR-, RAS- and c-MET-inhibitors on growth and metastatic colonization of HNSCC xenografts Subcutaneously growing HNSCC tumor xenografts ((A) PE/CA-PJ15, (B) PE/CA-PJ41) bearing mice were treated i.p. with the EGFR, Ras and c-MET inhibitors mono- and combination therapy as well. In the case of PJ15 cetuximab had no effect on tumor growth, but erlotinib and zoledronic acid decreased significantly the tumor volume either in monotherapy or in combination treatment. However, cetuximab had profound effect on tumor growth of PJ41 xenograft: all the investigated cetuximab-containing therapeutic regimes decreased dramatically the tumor volume. (C–D) Immunohistochemical detection of tumor cells using cytokeratin antibody in spleen (upper row) and liver (lower row) after colonization assay. In the case of cetuximab-resistant PJ15 (C) SU11274 treatment (right pictures in the panel) significantly decreased both the primary tumor mass and the number of tumor colonies in the liver compared to control (left pictures). The primary tumor mass was decreased to 20% of the control in treated animals, and the number of the liver colonies were decreased to less than 40% of the control group (E). However, SU11274 had no effect on either the primary tumor growth or liver colonization of cetuximab-sensitive PJ41 xenografts (F). Graph represent means ± SEM. Significance was measured by Mann-Whitney U-test. “C”: cetuximab; “E”: erlotinib, “Z”: zoledronic acid; “SU”: SU11274.
In Vivo Cetuximab Resistance Is Accompanied by a Profound In Vivo Sensitivity to the c-MET Specific Tyrosine Kinase Inhibitor SU11274
Given that c-MET and EGFR signaling pathways share molecular nodes (PI3K/Akt, MAPK), and recent data show the possible compensatory function of these receptor tyrosine kinase stimulated pathways [25], we examined the in vivo effect of the c-MET inhibitor SU11274 on primary tumor growth and liver colonization ability of the cetuximab resistant PE/CA-PJ15 and the cetuximab sensitive PJ41 HNSCC cells. Twenty-eight days after intrasplenic inoculation of tumor cells SCID mice were treated with SU11274 daily for 4 weeks. The effect of SU11274 treatment was monitored immunohistochemically using the epithelial cell-specific human cytokeratin antibody. SU11274 treatment significantly decreased primary tumor mass and even more dramatically reduced the number of hepatic tumor colonies in the case of the cetuximab resistant PE/CA-PJ15 cells (Figures 3A,C,E). However, the c-MET inhibitor did not have any significant effect either on primary tumor growth, or on liver colonization ability of the cetuximab sensitive PE/CA-PJ41 cancer cells (Figures 3B,D,F).
Expression and Activation Status of EGFR and c-MET in PE/CA-PJ15 and PE/CA-PJ41 Cells
Since the amount of plasma membrane-localized EGFR fundamentally influences the effectiveness of erlotinib and cetuximab treatments, we investigated receptor expression first at the DNA level. Based on FISH results, we found that both cell lines expressed normal EGFR gene copy number as we could not detect either gene amplification, or polysomy (see Supplementary Table S2). Next, we measured EGFR mRNA expression by quantitative real-time PCR detecting a 2-fold increase in receptor expression in PE/CA-PJ41 cells compared to the PJ15 cell line (Figure 4A). To check whether this difference is also manifested at the protein level, the ratio of EGFR positive cells and the level of expression were analyzed by flow cytometry and immunocytochemistry. We detected similar protein expression in PE/CA-PJ15 and PJ41 cells stained with an intracellular domain-specific EGFR antibody. However, the fluorescence intensity was weaker in PJ15 cells harboring the R521K EGFR mutation compared to PJ41 in the case of the extracellular domain-specific labeling (Table 2; Figure 4B). Moreover, we observed that 90% of the cells were positive for phospho-EGFR (Tyr1068) in both cell lines and even the expression level was found to be similar (Table 2; Figure 4C). These results raised the possibility that not the amount of the receptor is responsible, but the binding efficiency of the extracellular epitope-specific antibody differs between PE/CA-PJ15 and PJ41 cells. c-MET expression was also evaluated by the above methods, and we found that the percentage of the positive cells, expression level and activity were significantly higher in case of PE/CA-PJ15 cells compared to PJ41 (Table 2; Figures 4D,E).
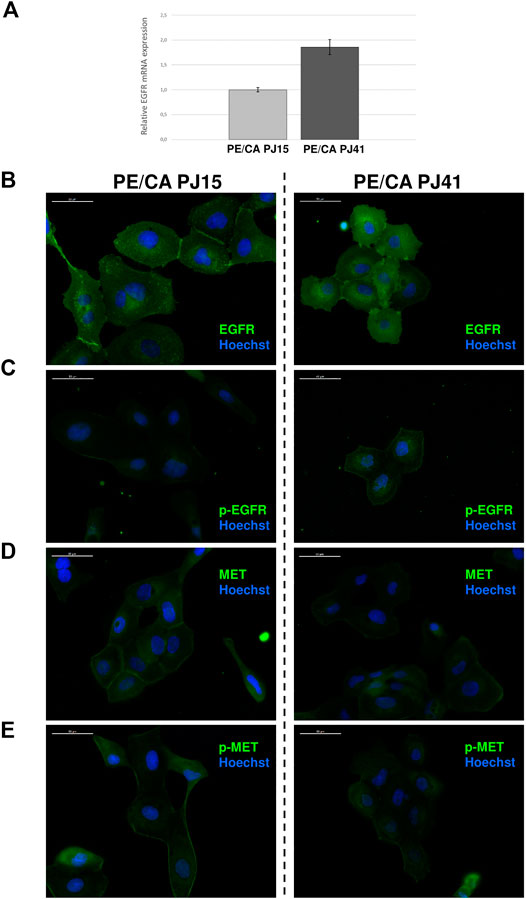
FIGURE 4. Expression of EGFR and c-MET in HNSCC cell lines. EGFR mRNA expression measured by quantitative real-time PCR was nearly 2-fold higher in PE/CA-PJ41 cells compared to the PJ15 cell line (A). Immunocytochemistry of PJ15 (left) and PJ41 (right) showed that both HNSCC cell lines express the EGFR (B) and c-MET (D) proteins. Moreover, these receptors are active without exogenous ligand activation in these cells detected by phospho-specific antibodies recognizing the active receptors (C,E). However, while EGFR (in line with the flow cytometric data in Table 2) expression is higher in PJ41 cells (B), c-MET and p-c-MET expression was slightly higher in PJ15 cells (D,E). White bars mark 50 μm distance.
Effect of EGFR, RAS and c-MET Inhibition on EGFR and ERK Activation in PE/CA-PJ15 and PE/CA-PJ41 Cells
To investigate whether the suggested decreased efficiency of cetuximab binding to the R521K mutant extracellular domain of EGFR in PE/CA-PJ15 cells results in a less effective inhibition of EGFR phosphorylation, we monitored the level of phospho-EGFR and phospho-ERK in HNSCC cells by immunoblots. Interestingly, we found that cetuximab treatment effectively decreased EGFR and ERK activity in both cell lines even at low concentration of 10 µM. Moreover, the inhibition effect was comparable to that of erlotinib treatment, and was more pronounced in PE/CA-PJ15 cells (Figure 5). These findings suggested that the in vivo sensitivity of HNSCC cells to cetuximab is not strictly related to the disruption of the tumor cell proliferation-supporting activity of the EGFR/ERK pathway. Our results raise the possible role of other RTK-stimulated pathways, and the involvement of the anti-EGFR antibody induced cytotoxic immune response in tumor elimination.
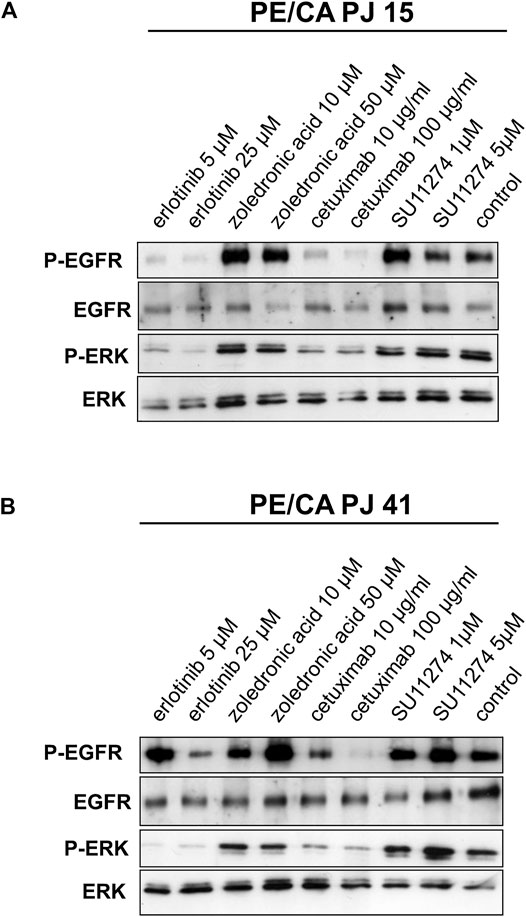
FIGURE 5. Effect of EGFR, RAS and c-MET inhibition on EGFR and ERK activity in PE/CA-PJ15 and PE/CA-PJ41 cells. Cetuximab treatment effectively decreased EGFR and ERK activity in both cell lines. The signaling activity was measured by Western blot using p-EGFR and p-ERK antibodies. The level of inhibition was comparable to the effect of erlotinib treatment and was more pronounced in PE/CA-PJ15 cells. The total EGFR and ERK labeling served as loading control.
Discussion
EGFR overexpression and mutation have been described as an oncogenic driver in several tumor types including HNSCC, making it a promising target for anticancer therapies in the last decade. Clinical trials showed that in patients with locally advanced HNSCC, cetuximab in combination with radiotherapy significantly increases the progression-free and overall survival of the patients [10]. Another clinical trial compared platinum-based plus fluorouracil combination chemotherapy with cetuximab or alone among patients with recurrent or metastatic squamous-cell carcinoma of the head and neck region. The result was similar: overall and progression-free survival were improved with cetuximab-combined concomitant chemotherapy [11].
However, additional targeted therapy improves clinical outcome only in a limited part of the patients, and lack of durable effect limits the clinical benefit of cetuximab in HNSCC patients [26].
Several studies have examined the possible causes of the primary and acquired resistance of EGFR blockade, and their findings suggest that not only the EGFR gene alterations alone, but many other signaling pathways play important role in the resistance to anti-EGFR therapy [27].
In our study, we examined two HNSCC cell lines to investigate possible causes of the failure of targeted therapy. Molecular biological analysis of EGFR ECD showed that four SNPs were found in the HNSCC cell lines. One of them is a guanine to adenine mutation, which causes an arginine to lysine amino acid change at codon 521 (c1808, Arg521Lys), leading to the EGFR R521K heterozygous polymorphism in case of PJ15 cells.
During in vitro proliferation assays, we measured significant inhibition on both PE/CA-PJ41 and PJ15 cells using the EGFR specific TK inhibitor erlotinib and the RAS inhibitor zoledronate, but interestingly, cetuximab, already used in clinical practice, was ineffective, suggesting that the mechanism of action underlying cetuximab therapy might be different than direct cell proliferation inhibition, or apoptosis induction.
In the combination treatment experiments, erlotinib and zoledronate in combination had the most potent antiproliferative effect, but the additional combination with cetuximab could not suppress cell proliferation in either cell lines any further.
We could further demonstrate the in vitro ineffectiveness of cetuximab: in the drug/antibody induced apoptosis assay cetuximab treatment did not induce apoptosis either in PJ15, or PJ41 cell cultures. Also, in line with the results of the in vitro proliferation assays, the treatment with TK inhibitor erlotinib and RAS inhibitor zoledronate caused significant cell death in both cell lines. Furthermore, we observed that the c-MET inhibitor SU11274 provoked remarkable apoptosis in PJ15 cells (harboring the R521K EGFR polymorphism), while it was ineffective in the homozygous wild type PE/CA-PJ41 cells.
Cetuximab is a monoclonal antibody and has a dual mechanism of action in vivo. Primarily, it binds the extracellular ligand-binding domain of the EGF receptor, thereby blocking the transmission of information through the specific signaling pathway and evokes a cascade of complex antitumor effects, including cell-cycle arrest, induction of cell death, inhibition of angiogenesis and metastasis, and downregulation of EGF receptor expression [28]. Besides, there are other publications assuming that cetuximab binding induces various immune interactions via its Fc fragment. The tumor cells with bound cetuximab antibodies are more easily detected by NK cells, and other nonspecific cytotoxic cells which leads to antibody-dependent cellular cytotoxicity (ADCC). In addition, binding the components of complement cascade through the Fc region, cetuximab may initiate complement-dependent cytotoxicity (CDC) as well [29,30].
In our in vivo experiments, SCID mice bearing PE/CA-PJ15-and PJ41-derived xenografts were treated with specific targeted inhibitors, such as erlotinib, zoledronate and cetuximab. Unlike in our in vitro assay models, the two HNSCC cell lines showed significantly different sensitivity to cetuximab in vivo. In case of PE/CA-PJ41 cells harboring the wild type EGFR, cetuximab showed dramatic inhibitory effect on the tumor growth: tumors were completely eradicated upon treatment with all combined therapeutic combination containing the monoclonal antibody. Nevertheless, PE/CA-PJ15 cells, expressing the R521K mutant variant of the EGF receptor, showed no response to cetuximab treatment, in line with our in vitro findings. Cetuximab barely affected tumor growth, while the co-treatment with erlotinib and zoledronate decreased tumor proliferation significantly. The effect of erlotinib and zoledronate in combination could not be achieved by any other therapeutic regimens.
Similar observations were made in flow cytometric and immunohistochemical experiments, since we detected nearly the same EGFR protein expression in PE/CA-PJ15 and PJ41 cells with the IC domain specific antibody, but the fluorescent intensity with the EC domain specific antibody was significantly weaker in the mutant cell line than in PJ41. Moreover, we found that cetuximab treatment effectively reduced the level of phospho-EGFR and phospho-ERK in both cell lines on immunoblots. This also means that, despite the decreased efficiency of cetuximab binding, PE/CA-PJ15 cells with the R521K mutant receptor still undergo the inactivation of EGFR activity.
These results raised the possibility that EGFR R521K polymorphism acts for an important mechanism of intrinsic resistance to cetuximab therapy, since it causes decreased binding efficiency of the extracellular epitope specific antibody. These findings also suggest the involvement of the anti-EGFR antibody induced cytotoxic immune response in tumor elimination and that multi-targeting approach to the EGFR signaling cascade may eliminate cetuximab resistant tumors.
Recently, numerous studies have indicated that activation of alternative pathways and receptors might be the reason of the acquired failure of anti-EGFR therapy. Many pre-clinical studies have described that HER family receptors are compensatory overexpressed when EGFR is blocked, leading to therapy resistance [17]. Another study demonstrated that HER3 receptor is upregulated and activated in HNSCC cells after cetuximab therapy, which negatively influences the response to mAb treatment [31]. Activation of HER2 and HER4 receptors has been also reported as a potential key player in the mechanism of cetuximab resistance. Their signaling pathways share downstream effectors with EGFR, by which HNSCC cells could escape form cetuximab inhibition [17,32]. Due to extensive crosstalk among HER receptors, targeting multiple members of the HER receptor family with pan-ErbB inhibitors (dacomitinib, afatinib) could reach explicit therapeutic benefit and overcome resistance to EGFR targeted therapies [32,33].
Besides HER family and its downstream signaling proteins, in the presence of EGFR blockade, activation of several alternative growth factor receptor pathways has been reported in recent years, such as ALK, VEGF, IGF-1, MET [25,34,35]. In our extended experiments with the specific c-MET inhibitor SU11274, we found that it effectively decreases in vitro proliferative capacity of both cell lines. Moreover, we observed that this TK inhibitor provoked remarkable apoptosis in PJ15 cells harboring the R521K EGFR polymorphism, while it was ineffective in the homozygous wild type PE/CA-PJ41 cells.
Based on our immunofluorescent and flow cytometric assays, we can assess that the c-MET receptor was expressed by both cell lines and was active without the presence of its extracellular ligand, but the phosphorylated c-MET signal in the PJ15 cell line was significantly more active compared to the other cell line. This result coincides with the observation [17] that c-MET receptor is present in constitutively activated form in cetuximab resistant cells.
Since aberrant c-MET function plays an important role in the metastatic capacity, we examined the in vivo effect of the c-MET inhibitor SU11274 on primary tumor proliferation and liver colonization ability of the cetuximab resistant PE/CA-PJ15 and the cetuximab sensitive PJ41 HNSCC cells. In the PE/CA-PJ15 cell line the c-MET inhibitor significantly reduced primary tumor growth and even more decreased hepatic colonization. In contrast, in the cetuximab sensitive PE/CA-PJ41 cell line, we could not observe significant effect either on the size of primary tumor mass, or on liver colonization ability.
Taken together, the HNSCC cell line expressing the R521K mutant form of the EGF receptor did not respond well to cetuximab treatment. Prior to initiating antibody therapy in the clinical practice, it would be beneficial to detect the presence of the R521K polymorphism, as it may allow to identify a substantial group of the patients with intrinsic resistance to the monoclonal antibody. However, this connection should be further investigated to see whether R521K polymorphism leads to cetuximab resistance. A recent study found that both in vivo xenografts and clinical samples follow the pattern suggested [18].
Based on our results, tumors that are only moderately sensitive to anti-EGFR antibody therapy can respond well to c-MET receptor targeting, but in the case of wild-type EGFR-expressing HNSCCs, there is a major role in signal transmission through the EGF receptor, the anti-EGFR antibody therapy seems mostly effective.
New therapeutic strategies such as inhibitory combinations or the use of multi-targeting inhibitors may provide a breakthrough in the treatment of cetuximab-resistant tumors. Also, combination therapy of EGFR inhibitors and c-MET inhibitors is a promising way to enhance in vivo and clinical response to targeted therapy in advanced HNSCC, rising new possibility to patients in need of an efficient therapy.
Conclusions
As EGFR-targeting treatments in advanced HNSCC patients often fail to express antitumor activity, there is an urge to select patients eligible for anti-EGFR therapy and create novel modalities for those who are not. Based on our in vitro and in vivo results, EGFR R521K polymorphism can be a potential predictive marker for cetuximab therapy response. Importantly, c-MET-targeted therapy might be the key to successfully achieve clinical response of the tumors showing resistance to EGFR-targeted therapies.
Data Availability Statement
The raw data supporting the conclusion of this article will be made available by the authors, without undue reservation.
Ethics Statement
All animal housing and breeding processes (permission No: PEI/001/1738-3/2015) and animal experiments (permission No: PEI/001/2574-6/2015) were conducted following standards and procedures approved by the Animal Care and Use Committee of the National Institute of Oncology, Budapest.
Author Contributions
This research has been conceived and designed by JT, IK, and MC with valuable inputs from all other authors. Research resources were provided by JT Supervision of experiments by JT and B.S.1; SU11274 were synthesized and characterized by B.S.2 and LÅ; cell culturing and in vitro cell viability assays were done by GAN, AK, and ZH; real-time qPCR, WB and flow cytometry studies were performed by MC, VL, DT, JD. ER, TB; anti-tumor and anti-metastatic in vivo studies were performed and evaluated by GAN, AK, IK, and JT; ex vivo studies and evaluation were carried out by GAN and AK, the manuscript was written by GAN, DT, MC, and JT. All authors reviewed and corrected the manuscript.
Funding
This research was funded by the National Research, Development and Innovation Office (K116295 JT), Hungary. Financial support of the molecular biology lab from the 2019 Thematic Excellence Program (TUDFO/51757/2019-ITM and TKP2020-NKA-26) is greatly acknowledged.
Conflict of Interest
BS, JD, and LŐ were employed by Vichem Chemie Ltd.
The authors declare that the research was conducted in the absence of any commercial or financial relationships that could be construed as a potential conflict of interest.
Acknowledgments
The authors would like to acknowledge Katalin Parragné Derecskei for the help in ex vivo studies, and Anita Hidvégi, Irén Bodrogi Mayer and Anna Mária Tóth for the help with in vivo experiments.
Supplementary Material
The Supplementary Material for this article can be found online at: https://www.por-journal.com/articles/10.3389/pore.2021.620256/full#supplementary-material.
Abbreviations
AEC, 3-amino-9-ethylcarbazole; ATCC, american type culture collection; c-MET, mesenchymal epithelial transition factor; dNTP, deoxy-nucleotide-triphosphate; ECACC, European collection of cell cultures; EGFR, epidermal growth factor receptor; FDA, food and drug administration; FITC, fluorescein-5-isothiocyanate; HNSCC, head and neck squamous cell carcinoma; IC domain/EC domain, intracellular domain/extracellular domain; KRAS, Kirsten rat sarcoma 2 viral oncogene homolog; NSCLC, non-small-cell lung carcinoma; PCR, polymerase chain reaction; RTK, receptor tyrosine kinase; SNP, single nucleotide polymorphisms; TKI, tyrosine kinase inhibitor
References
1. Marur, S, and Forastiere, AA Head and Neck Cancer: Changing Epidemiology, Diagnosis, and Treatment. Mayo Clinic Proceedingsáprilis (2008) 83(4):489–501. doi:10.4065/83.4.489
2. Döbrossy, L Epidemiology of Head and Neck Cancer: Magnitude of the Problem. Cancer Metastasis Rev (2005) 24(1):9–17. doi:10.1007/s10555-005-5044-4
3. Goerner, M, Seiwert, TY, and Sudhoff, H Molecular Targeted Therapies in Head and Neck Cancer—an Update of Recent Developements -. Head Neck Oncol (2010) 2:8. doi:10.1186/1758-3284-2-8
4. Mehra, R, Cohen, RB, and Burtness, BA The Role of Cetuximab for the Treatment of Squamous Cell Carcinoma of the Head and Neck. Clin Adv Hematol Oncoloktóber (2008) 6(10):742–50.
5. Zimmermann, M, Zouhair, A, Azria, D, and Ozsahin, M The Epidermal Growth Factor Receptor (EGFR) in Head and Neck Cancer: its Role and Treatment Implications. Radiat Oncol (2006) 1:11. doi:10.1186/1748-717x-1-11
6. Chung, CH, Ely, K, McGavran, L, Varella-Garcia, M, Parker, J, Parker, N, et al. Increased Epidermal Growth Factor Receptor Gene Copy Number Is Associated with Poor Prognosis in Head and Neck Squamous Cell Carcinomas. Jco (2006) 24(25):4170–4176. doi:10.1200/jco.2006.07.2587
7. Rogers, SJ, Harrington, KJ, Rhys-Evans, P, O-Charoenrat, P, and Eccles, SA Biological Significance of C-erbB Family Oncogenes in Head and Neck Cancer. Cancer Metastasis Rev (2005) 24(1):47–69. doi:10.1007/s10555-005-5047-1
8. Ali, MALS, Gunduz, M, Nagatsuka, H, Gunduz, E, Cengiz, B, Fukushima, K, et al. Expression and Mutation Analysis of Epidermal Growth Factor Receptor in Head and Neck Squamous Cell Carcinoma. Cancer Sci (2008) 99(8):1589–94. doi:10.1111/j.1349-7006.2008.00861.x
9. Temam, S, Kawaguchi, H, El-Naggar, AK, Jelinek, J, Tang, H, Liu, DD, et al. Epidermal Growth Factor Receptor Copy Number Alterations Correlate with Poor Clinical Outcome in Patients with Head and Neck Squamous Cancer. Jco (2007) 25(16):2164–70. doi:10.1200/jco.2006.06.6605
10. Bonner, JA, Harari, PM, Giralt, J, Azarnia, N, Shin, DM, Cohen, RB, et al. Radiotherapy Plus Cetuximab for Squamous-Cell Carcinoma of the Head and Neck. N Engl J Med (2006) 354(6):567–78. doi:10.1056/nejmoa053422
11. Vermorken, JB, Mesia, R, Rivera, F, Remenár, E, Kawecki, A, Rottey, S, et al. Platinum-based Chemotherapy Plus Cetuximab in Head and Neck Cancer. N Engl J Med (2008) 359:1116. doi:10.1056/NEJMoa0802656
12. Astsaturov, I, Cohen, R, and Harari, P EGFR-targeting Monoclonal Antibodies in Head and Neck Cancer. Ccdt (2006) 6(8):691–710. doi:10.2174/156800906779010191
13. Soulieres, D, Senzer, NN, Vokes, EE, Hidalgo, M, Agarwala, SS, and Siu, LL Multicenter Phase II Study of Erlotinib, an Oral Epidermal Growth Factor Receptor Tyrosine Kinase Inhibitor, in Patients with Recurrent or Metastatic Squamous Cell Cancer of the Head and Neck. Jco (2004) 22(1):77–85. doi:10.1200/jco.2004.06.075
14. Cohen, EEW, Rosen, F, Stadler, WM, Recant, W, Stenson, K, Huo, D, et al. Phase II Trial of ZD1839 in Recurrent or Metastatic Squamous Cell Carcinoma of the Head and Neck. Jco (2003) 21(10):1980–7. doi:10.1200/jco.2003.10.051
15. Cohen, EEW, Kane, MA, List, MA, Brockstein, BE, Mehrotra, B, Huo, D, et al. Phase II Trial of Gefitinib 250 Mg Daily in Patients with Recurrent And/or Metastatic Squamous Cell Carcinoma of the Head and Neck. Clin Cancer Res. 2005;11(23):8418–8424. doi:10.1158/1078-0432.ccr-05-1247
16. Wheeler, DL, Iida, M, Kruser, TJ, Nechrebecki, MM, Dunn, EF, Armstrong, EA, et al. Epidermal Growth Factor Receptor Cooperates with Src Family Kinases in Acquired Resistance to Cetuximab. Cancer Biol Ther (2009) 8(8):696–703. doi:10.4161/cbt.8.8.7903
17. Wheeler, DL, Huang, S, Kruser, TJ, Nechrebecki, MM, Armstrong, EA, Benavente, S, et al. Mechanisms of Acquired Resistance to Cetuximab: Role of HER (ErbB) Family Members. Oncogene (2008) 27(28):3944–56. doi:10.1038/onc.2008.19
18. Braig, F, Kriegs, M, Voigtlaender, M, Habel, B, Grob, T, Biskup, K, et al. Cetuximab Resistance in Head and Neck Cancer Is Mediated by EGFR-K521 Polymorphism. Cancer Res (2017) 77(5):1188–1199. doi:10.1158/0008-5472.can-16-0754
19. Benavente, S, Huang, S, Armstrong, EA, Chi, A, Hsu, K-T, Wheeler, DL, et al. Establishment and Characterization of a Model of Acquired Resistance to Epidermal Growth Factor Receptor Targeting Agents in Human Cancer Cells. Clin Cancer Res (2009) 15(5):1585–92. doi:10.1158/1078-0432.ccr-08-2068
20. Boerner, JL Role of Src Family Kinases in Acquired Resistance to EGFR Therapies in Cancer. Cancer Biol Ther (2009) 8(8):704–6. doi:10.4161/cbt.8.8.8183
21. Koppikar, P, Choi, S-H, Egloff, AM, Cai, Q, Suzuki, S, Freilino, M, et al. Combined Inhibition of C-Src and Epidermal Growth Factor Receptor Abrogates Growth and Invasion of Head and Neck Squamous Cell Carcinoma. Clin Cancer Res (2008) 14(13):4284–91. doi:10.1158/1078-0432.ccr-07-5226
22. Cortesina, G, Martone, T, Galeazzi, E, Olivero, M, De Stefani, A, Bussi, M, et al. Staging of Head and Neck Squamous Cell Carcinoma Using theMET Oncogene Product as Marker of Tumor Cells in Lymph Node Metastases. Int J Cancer (2000) 89(3):286–92. doi:10.1002/1097-0215(20000520)89:3<286::aid-ijc12>3.0.co;2-u
23. Seiwert, TY, Jagadeeswaran, R, Faoro, L, Janamanchi, V, Nallasura, V, El Dinali, M, et al. The MET Receptor Tyrosine Kinase Is a Potential Novel Therapeutic Target for Head and Neck Squamous Cell Carcinoma. Cancer Res (2009) 69(7):3021–31. doi:10.1158/0008-5472.can-08-2881
24. Sattler, M, Pride, YB, Ma, P, Gramlich, JL, Chu, SC, Quinnan, LA, et al. A Novel Small Molecule Met Inhibitor Induces Apoptosis in Cells Transformed by the Oncogenic TPR-MET Tyrosine Kinase. Cancer Res (2003) 63(17):5462–9.
25. Madoz-Gúrpide, J, Zazo, S, Chamizo, C, Casado, V, Caramés, C, Gavín, E, et al. Activation of MET Pathway Predicts Poor Outcome to Cetuximab in Patients with Recurrent or Metastatic Head and Neck Cancer. J Transl Med (2015) 13:282. doi:10.1186/s12967-015-0633-7
26. Cohen, RB Current Challenges and Clinical Investigations of Epidermal Growth Factor Receptor (EGFR)- and ErbB Family-Targeted Agents in the Treatment of Head and Neck Squamous Cell Carcinoma (HNSCC). Cancer Treat Rev (2014) 40(4):567–77. doi:10.1016/j.ctrv.2013.10.002
27. Zhao, B, Wang, L, Qiu, H, Zhang, M, Sun, L, Peng, P, et al. Mechanisms of Resistance to Anti-EGFR Therapy in Colorectal Cancer. Oncotarget (2017) 8(3):3980–4000. doi:10.18632/oncotarget.14012
28. Bou-Assaly, W, and Mukherji, S Cetuximab (Erbitux). AJNR Am J Neuroradiol (2010) 31(4):626–7. doi:10.3174/ajnr.a2054
29. Holubec, L, Polivka, J, Safanda, M, Karas, M, and Liska, V The Role of Cetuximab in the Induction of Anticancer Immune Response in Colorectal Cancer Treatment. Ar (2016) 36(9):4421–6. doi:10.21873/anticanres.10985
30. Trotta, AM, Ottaiano, A, Romano, C, Nasti, G, Nappi, A, De Divitiis, C, et al. Prospective Evaluation of Cetuximab-Mediated Antibody-dependent Cell Cytotoxicity in Metastatic Colorectal Cancer Patients Predicts Treatment Efficacy. Cancer Immunol Res (2016) 4(4):366–74. doi:10.1158/2326-6066.cir-15-0184
31. Wang, D, Qian, G, Zhang, H, Magliocca, KR, Nannapaneni, S, Amin, ARMR, et al. HER3 Targeting Sensitizes HNSCC to Cetuximab by Reducing HER3 Activity and HER2/HER3 Dimerization: Evidence from Cell Line and Patient-Derived Xenograft Models. Clin Cancer Res (2017) 23(3):677–86. doi:10.1158/1078-0432.ccr-16-0558
32. De Pauw, I, Lardon, F, Van den Bossche, J, Baysal, H, Fransen, E, Deschoolmeester, V, et al. Simultaneous Targeting of EGFR , HER 2, and HER 4 by Afatinib Overcomes Intrinsic and Acquired Cetuximab Resistance in Head and Neck Squamous Cell Carcinoma Cell Lines. Mol Oncol (2018) 12(6):830–54. doi:10.1002/1878-0261.12197
33. Ather, F, Hamidi, H, Fejzo, MS, Letrent, S, Finn, RS, Kabbinavar, F, et al. Dacomitinib, an Irreversible Pan-ErbB Inhibitor Significantly Abrogates Growth in Head and Neck Cancer Models that Exhibit Low Response to Cetuximab. PLoS One (2013) 8(2):e56112. doi:10.1371/journal.pone.0056112
34. Adkins, D, Mehan, P, Ley, J, Siegel, MJ, Siegel, BA, Dehdashti, F, et al. Pazopanib Plus Cetuximab in Recurrent or Metastatic Head and Neck Squamous Cell Carcinoma: an Open-Label, Phase 1b and Expansion Study. Lancet Oncol (2018) 19(8):1082–93. doi:10.1016/s1470-2045(18)30350-4
35. Ouyang, X, Barling, A, Lesch, A, Tyner, JW, Choonoo, G, Zheng, C, et al. Induction of Anaplastic Lymphoma Kinase (ALK) as a Novel Mechanism of EGFR Inhibitor Resistance in Head and Neck Squamous Cell Carcinoma Patient-Derived Models. Cancer Biol Ther (2018) 19(10):921–33. doi:10.1080/15384047.2018.1451285
Keywords: EGFR, c-Met, HNSCC, R521K, targeted tumor therapy
Citation: Nelhűbel GA, Cserepes M, Szabó B, Türk D, Kárpáti A, Kenessey I, Rásó E, Barbai T, Hegedűs Z, László V, Szokol B, Dobos J, Őrfi L and Tóvári J (2021) EGFR Alterations Influence the Cetuximab Treatment Response and c-MET Tyrosine-Kinase Inhibitor Sensitivity in Experimental Head and Neck Squamous Cell Carcinomas. Pathol. Oncol. Res. 27:620256. doi: 10.3389/pore.2021.620256
Received: 22 October 2020; Accepted: 01 April 2021;
Published: 03 May 2021.
Edited by:
Anna Sebestyén, Semmelweis University, HungaryCopyright © 2021 Nelhűbel, Cserepes, Szabó, Türk, Kárpáti, Kenessey, Rásó, Barbai, Hegedűs, László, Szokol, Dobos, Őrfi and Tóvári. This is an open-access article distributed under the terms of the Creative Commons Attribution License (CC BY). The use, distribution or reproduction in other forums is permitted, provided the original author(s) and the copyright owner(s) are credited and that the original publication in this journal is cited, in accordance with accepted academic practice. No use, distribution or reproduction is permitted which does not comply with these terms.
*Correspondence: József Tóvári, tozsi@oncol.hu