- 1Department of Hematology, Lanzhou University Second Hospital, Lanzhou, China
- 2Department of Endocrinology, Lanzhou University Second Hospital, Lanzhou, China
- 3Department of Hematology, The Affiliated Hospital of Southwest Medical University, Luzhou, China
Multiple myeloma (MM) is a hematologic disorder characterized by the accumulation of malignant plasma cells in the bone marrow. Genetic and environmental factors are contributed to the etiology of MM. Notably, studies have shown that obesity increases the risk of MM and worsens outcomes for MM patients. Adipokines play an important role in mediating the close association between MM and metabolic derangements. In this review, we summarize the epidemiologic studies to show that the risk of MM is increased in obese. Accumulating clinical evidence suggests that adipokines could display a correlation with MM. In vitro and in vivo studies have shown that adipokines are linked to MM, including roles in the biological behavior of MM cells, cancer-associated bone loss, the progression of MM, and drug resistance. Current and potential therapeutic strategies targeted to adipokines are discussed, proposing that adipokines can guide early patient diagnosis and treatment.
Introduction
Multiple myeloma (MM) is a blood cancer of abnormal clonal plasma cells in the bone marrow. MM accounts for 1% of neoplastic diseases and is the second most common hematological malignancy that commonly affects older adults (median age at diagnosis is 69 years) [1, 2]. Patients often suffer from anemia, kidney injury, bone destruction, and hypercalcemia. MM develops from the premalignant state monoclonal gammopathy of undetermined significance (MGUS) and smoldering multiple myeloma (SMM). To diagnose MM, clinical symptoms are used, and the monoclonal proteins in blood and urine need to be detected. Clinical symptoms are used to diagnose MM, and the monoclonal proteins in blood and urine must be detected. Autologous stem-cell transplantation (ASCT), proteasome inhibitors, immunomodulatory drugs, and monoclonal antibodies have improved outcomes with MM. Although therapeutic advances made in the past few years have led to improved outcomes and longer survival, MM remains incurable. Therefore, it is of great significance to study the etiology and pathogenesis of myeloma to find new therapeutic targets and improve the survival rate of patients.
Although little is known about the etiology of MM, genetic, antigenic stimulation, and environmental factors are believed to contribute to MM onset and progression [3]. Some risk factors are associated with MM, such as obesity, male sex, age, chronic inflammation, and dioxin exposure [2]. Accumulating evidence suggests that obesity plays a critical role in the risk of developing MM, and the increased body mass index (BMI) has been linked to the progression and higher mortality [4–6]. The bone marrow microenvironment plays a vital role in the proliferation, survival, progression, and drug resistance of MM cells [2]. The bone marrow microenvironment is composed of cellular and non-cellular compartments. The cellular compartment comprises MM cells, lymphocytes, natural killer cells, macrophages, monocytes, dendritic cells, osteoclasts, osteoblasts, and adipocytes. And the non-cellular compartment is made up of extracellular matrix proteins, adhesion molecules, cytokines, and growth factors. Bone marrow adipocytes are the main component of the bone marrow microenvironment [7]. Bone marrow adipocytes in close contact with bone cells, hematopoietic cells, and immune cells are considered within bone metabolism, hematopoiesis, cancer, and systemic energy metabolism. Adipocytes found in bone marrow have important connections with bone cells, hematopoietic cells, and immune cells. These connections play significant roles in bone metabolism, hematopoiesis, cancer, and systemic energy metabolism. Using the C57BL/KaLwRij murine model of myeloma, mice were inoculated with 5TGM1-GFP cells or PBS control by intravenous injection. Bone marrow adipocytes were found to be increased in early-stage myeloma [8]. MM cell lines (RPMI-8226 and NCI-H929) were cocultured with adipocytes and showed greater capabilities of proliferation and adhesion [9]. These findings indicate that bone marrow adipocytes promote the proliferation of MM cells.
Obesity is closely related to the onset of many types of malignancies [10]. The mechanisms linking obesity to cancer involve insulin resistance, abnormalities of the IGF-1 axis, inappropriate sex hormone secretion, inflammation and oxidative stress, adipokines action, microenvironment perturbations, and the altered intestinal microbiome [11]. In obesity, adipose tissue hypoxia triggers alternations of adipokines levels altering adipokines levels, may be associated with the progression of various cancers [12–14]. This review critically summarizes the rapidly expanding field of bone marrow adipocytes as an endocrine organ and how they communicate with MM cells through the secretion of adipokines. First, we will briefly address the changes in adipokines discuss the changes in adipokines of MM. Second, we will present the biological effect of adipokines. Finally, we will elaborate on the adipokines associated with MM progression as growth, proliferation, and drug resistance, including findings from in vitro, in vivo, and human studies, and therapeutic strategy target to adipokines.
Adipokines in MM: friend or foe?
Adipose tissue was initially thought of as a fat depot but is now widely recognized as an endocrine organ that secretes numerous peptide factors called adipokines. They play an essential role in metabolic diseases and neoplasms in paracrine and endocrine. They can regulate glucose and lipid metabolism, inflammation, and immune response [15]. After the first adipokine leptin was discovered, over 600 adipokines have been discovered and studied [16]. Some adipokines stimulate cancer progression through oncogenic signaling or indirect mechanisms, such as angiogenesis and immunomodulation [17], while others with oncogenic effects have their expression suppressed in malignancies. Therefore, alterations in adipokines may affect the processes and the immune responses of cancers. The role of adipokines in MM is comparatively less known. A selected group of adipokines with demonstrated roles in MM are reviewed below (Figure 1).
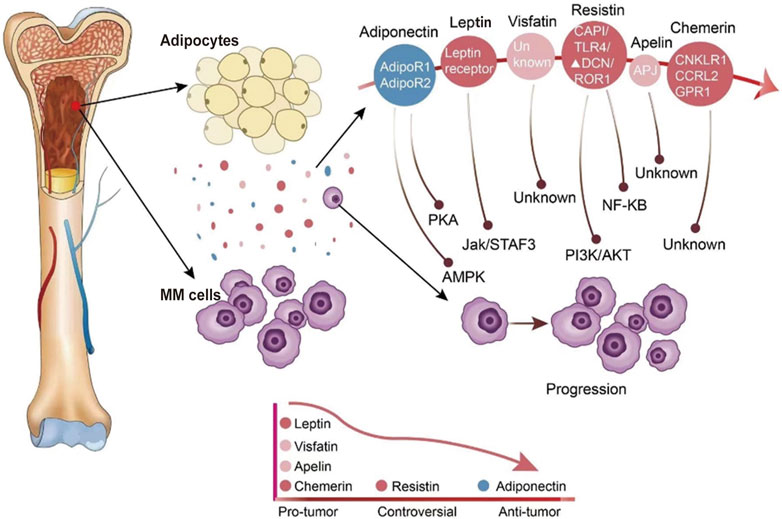
FIGURE 1. The expression of adipokines in the bone marrow environment and adipokines modify the behavior of MM cells. Adipocytes in the bone marrow secrete a variety of different adipokines. The expression of adipokines in the bone marrow microenvironment of MM patients is indicated by different colors, with blue indicating reduced secretion and red indicating increased secretion.
Adiponectin
Adiponectin is a 28 KDa protein secreted by adipose tissue, which is one of the most widely studied adipokines [18]. Besides the adipose tissue, adiponectin is also produced to some extent by bone marrow [19]. Adiponectin has two major receptors, AdipoR1 and AdipoR2. A third receptor had been isolated, identical to a unique cadherin molecule [20, 21]. Adiponectin binds to receptors to participate in insulin-sensitizing, lipid metabolism, energy regulation, inflammation, and cancer development [22]. Circulating levels of adiponectin show an inverse correlation with multiple diseases, such as obesity, type 2 diabetes, atherosclerosis, hypertension, dyslipidemia, and nonalcoholic fatty liver disease [23–27]. It also negatively correlates with several cancers, one of which is MM [28, 29]. Thus adiponectin has generally been considered a beneficial adipokine.
In a pooled investigation of 624 MM patients and 1,246 controls from seven cohorts, Hofmann et al. revealed that serum adiponectin levels were lower among the patients than controls (medians of 11.5 and 12.8 μg/mL, respectively; p = 0.001). Furthermore, they stratified the samples according to BMI and observed an inverse association between adiponectin levels and MM risk among overweight (OR = 0.41, CI = 0.26–0.65, p < 0.001) or obese subjects (OR = 0.41, CI = 0.17–0.98, p ≤ 0.039) [30]. Hofmann et al. investigated the levels of adiponectin in serum samples from 213 patients (84 with MGUS, 104 with SMM, and 25 with MM). They found total adiponectin levels were 16% lower among SMM patients (CI = −31% to 2%) and 20% lower among MM patients (CI = −40% to 7%) compared to patients with MGUS [29]. Furthermore, another study showed decreased levels of high molecular weight adiponectin in MGUS patients who progressed to MM (4.5 ± 0.5 μg/mL) compared to MGUS who did not progress to MM (6.4 ± 1.6 μg/mL). The studies used gene array analysis of bone marrow between KaLwRij mice that permit murine 5T myeloma cells compared to nonpermissive mice. Adiponectin was decreased in the bone marrow of KaLwRij mice [31]. These studies suggested low adiponectin levels may associate with MM, particularly among overweight and obese individuals. And adiponectin may play a protective role in the development of MGUS to SMM/MM. This suggests that adiponectin may have a protective effect in the development of MGUS to SMM/MM. Lower blood levels of adiponectin in MM patients before the start of any treatment suggest that adiponectin represents a potential biomarker at the onset of disease to predict progression.
The mechanisms behind the association between adiponectin and MM are still unclear. Some studies provide insights into the mechanisms of adiponectin in MM. Adiponectin induces the apoptosis and cell cycle arrest of MM cells via activation of protein kinase A (PKA) and increased AMP-activated protein kinase (AMPK) activation [32]. Furthermore, CD169+ radiation-resistant tissue-resident macrophages regulate MM cells in the bone marrow via interleukin-6(IL-6) and tumor necrosis factor α (TNF-α) pathway [33]. And adiponectin Adiponectin receptor signaling suppresses TNF-α expression by T cells or myeloid cells [34]. Therefore, we can speculate whether adiponectin changes the biological effect of MM cells by affecting the inflammatory factors. The correlation between the effects of adiponectin receptors and MM progression remains to be studied. Interestingly, adiponectin was found to be downregulated by MM cells themselves via the blockade of TNF-α [8]. It is suggested that adiponectin interacts with myeloma cells to alter the microenvironment of the disease. In addition, it is well known that MM cells enhance the process of osteoclastogenesis and bone resorption while suppressing osteoblast cells differentiation, leading to systemic bone destruction with rapid bone loss [35]. Some studies have paid attention to the association between adiponectin and bone disease. Serum level of adiponectin correlated with markers of bone disease, such as OCN, CTX, and PINP. In vitro study, the anti-osteolysis effects of adiponectin may be explained by the inhibition of osteoclasts via the mTOR signaling pathway [36]. In murine models of MM, adiponectin suppressed nerve growth factor, which is thought to be associated with bone pain [37]. Adiponectin may have a positive effect on myeloma bone disease. In summary, adiponectin exerts its antitumor effects in MM by promoting cell apoptosis, causing cell cycle arrest, inhibiting osteolysis, and possibly modulating cytokines in the bone marrow microenvironment (Table 1).
These beneficial effects of adiponectin have promoted research on adjuvants that mimic adiponectin or the adiponectin receptor agonist to treat MM. L-4F is an apolipoprotein mimetic peptide that can upregulate the adiponectin level [38]. Moreover, L-4F affected inhibiting the progression of MGUS to MM [31]. In addition, L-4F, downregulating the expression of nerve growth factor (NGF) and IL-6, has a positive effect on destructive osteolytic bone disease [37]. The adiponectin receptor agonist adipoRon can inhibit MM by reversing the effect of TNF-α and IL-6 [37]. Since it was found that adiponectin-induced apoptosis in MM cells may be achieved by downregulating acetyl-CoA-carboxylase. 5-(tetradecyloxy)-2-furan carboxylic acid (TOFA), an acetyl-CoA-carboxylase inhibitor, was found to inhibit fatty acid synthesis and also can inhibit MM cell proliferation [32]. Taken together, the anti-myeloma effect of adiponectin may be a promising target candidate to start with the new area of MM.
Leptin
Leptin was the first adipokine to be identified in 1994 and was shown to be expressed in adipose tissue as a secreted 16 kDa polypeptide [39]. Leptin binds to the leptin receptor to exert pleiotropic effects, such as energy homeostasis, metabolism, hematopoiesis, and immunomodulation [40, 41]. A clinical study conducted by Considine et al. showed that serum leptin concentrations in obese subjects were higher than the normal weight subjects [42]. Emerging findings have demonstrated leptin levels in serum samples related to various cancers. Serum leptin level was higher in colorectal cancer patients than in healthy controls [43]. Similar results were found in breast cancer, prostate cancer, non-small cell lung cancer, and bladder cancer [44–47].
The assays of leptin levels in MM patients presented contradictory results. A study including 14 MM patients and 25 healthy controls demonstrated that the serum level of leptin was upregulated in the MM group (22.6 ± 14.7 ng/mL) compared to the healthy control (10.3 ± 7.6 ng/mL) [48]. Similarly, another research between newly diagnosed MM and healthy controls revealed higher serum leptin levels in MM patients [49]. A meta-analysis was conducted by Liu et al. in 2021 to analyze seven studies with 406 MM patients and 530 controls. They demonstrated higher leptin concentrations in MM patients than in controls (SMD = 0.87, CI = 0.33–1.41, z = 3.14, p = 0.002) [50]. Alexandrakis et al. examine examined leptin levels in the serum of 62 MM patients, according to the established Durie and Salmon criteria, they divided patients into stage I (n = 13), stage II (n = 22), stage III (n = 27). There was no significance according to each group. The increased leptin levels were not associated with the progression of MM [51]. These results indicate that leptin levels are increased in MM patients. However, Hofmann et al. investigated the serum levels of leptin in 174 patients (10.01 ± 2.64 ng/mL) and 348 controls (9.6 ± 2.71 ng/mL) between 1993 and 2001 in the US and found no significant difference (p = 0.78) [52].
The potential mechanisms of leptin on the development of MM could be largely as follows:The potential mechanisms of leptin in the development of MM could be as follows: (1) The pronounced proliferative response induced by leptin. Researchers cocultured MM cell line (RPMI-8226) with adipocytes, MM cells proliferated faster and displayed increased leptin protein level via pSTAT3/STAT-3 signaling [9]. Whether leptin promotes proliferation-mediated STAT3 signaling in RPMI-8226 must be verified by knockdown or by inhibiting leptin expression. Using the cell lines U266 and H929, researchers observed that leptin could promote proliferation, and they also found phosphorylated AKT and STAT3 proteins were increased when upregulated leptin [53]. (2) Leptin may promote MM development by inhibiting apoptosis. Leptin was shown to induce an upregulation of BCL-2 expression and the inhibition of caspase-3 activation [53]. It also can promote the expression of autophagic proteins via Jak/Stat3 pathway and then play an anti-apoptosis anti-apoptotic role in MM cells [54]. (3) Leptin as a regulator of anti-tumor immunity, may contribute to MM oncogenesis. Based on previous studies, we have known that invariant natural killer T (iNKT) cells with the effect of anti-tumor immunity were decreased in MM [55, 56]. Favreau et al. used the murine 5T33MM model, a pre-clinical immunocompetent model mimicking human MM disease, to investigate the leptin levels and expression of the leptin receptor. They found that the leptin level and expression of leptin receptors on iNKT cells were increased obviously. Similar results were obtained in the MM patients. In vitro, they coculture the MM cells with iNKT cells and found the IFN-γ production was inhibited. This effect can be reversed by leptin receptor antagonism [57]. Using a leptin-deficient mice model, Wang et al. found leptin increased CD8+T cell exhaustion and upregulated PD-1 expression, which impairs anti-tumor immunity [58] (Table 1).
The results of studies conducted to explore the associations between MM and leptin have been inconsistent and limited. Taken together, the vast majority of the above studies have concluded that leptin is highly expressed in MM. Myeloma types, different stages, risk stratification, and tumor heterogeneity may be responsible for the inconsistent results. Additionally, sex hormone secretion and sleep time could also affect leptin changes [59, 60]. Lastly, leptin levels are more influenced by the amount of subcutaneous fat than BMI [61]. Future clinical studies and additional mechanism studies of leptin in MM are needed further to clarify leptin’s alteration of the bone marrow microenvironment.
Visfatin
Visfatin, also known as nicotinamide phosphoribosyltransferase (NAMPT), was initially discovered as a protein for the differentiation of B cells. Named for pre-B-cell colony-enhancing factor (PBEF) [62]. In the intracellular, its function is mainly as the rate-limiting enzyme in NAD+ biosynthesis [63]. In the extracellular environment, it was thought to be an adipokine secreted by adipose tissue [64]. Visfatin is highly enriched in visceral fat and found in bone marrow, liver, kidney, and other tissues [62]. The main physiological functions are regulating metabolism, pro-inflammatory, and immune modulation [64]. Serum concentrations of visfatin were significantly higher in overweight and obedity obesity subjects when compared to normal weight controls [65]. Multiple studies conducted in the past decades investigated that visfatin was involved in the progression of different cancer types [66–69].
In MM, visfatin was shown to be elevated in 39 patients when compared with age-matched 20 healthy controls (102.76 ± 90.41 ng/mL vs. 22.55 ± 21.41 ng/mL; p < 0.001) [36]. Among some in vitro experiments, studies have tried to explore the mechanism of visfatin in MM researchers have attempted to investigate the mechanism of visfatin in MM. In one study, researchers using small interfering RNA (si-RNA) silenced the expression of visfatin then the growth and proliferation of MM cells were inhibited [70]. It was suggested that visfatin might upregulate NAD+ to supply energy for MM cells’ growth and survival. Apart from that, researchers also found that visfatin suppress apoptosis of MM cell depending on autophagy [71, 72]. Furthermore, visfatin can downregulate the sensibility of MM cells to bortezomib [73] and reduce the efficacy of anti-CD38 immunotherapies in MM [74]. Finally, in the SCID-rab model, inhibiting the expression of visfatin can suppress osteoclast formation and activity [75]. These studies suggest that visfatin regulates MM cells’ proliferation, apoptosis, drug sensitivity, and bone metastasis.
The research on visfatin as a promising target for treatment has yielded some results. FK866, an inhibitor of visfatin, enhanced the effect of bortezomib and reduced drug resistance. The mechanism of FK866 included activation of pro-apoptosis proteins, reduction of intracellular NAD+, and inhibition of angiogenesis [73].
Resistin
Resistin was discovered in 2001 and named for its function in promoting insulin resistance and glucose intolerance [76]. Resistin is secreted from white adipose adipose tissue in mice, whereas synthesized from monocytes and macrophages in humans. It is highly expressed in bone marrow [77]. Resistin is involved in insulin resistance, inflammation, immunoregulation, and cancer development [78]. Several studies have demonstrated that resistin can promote proliferation, associate with angiogenesis, regulate the epithelial to mesenchymal transition, and stimulate metastasis in various solid tumors [79–83]. Studies have yielded a positive correlations between serum levels of resistin and BMI [84]. Resistin has been shown to improve the survival and stemness of human breast cancer cells by activating STAT3 signaling [85]. At the neuronal levels, resistin bind to TLR4 receptors inducing the activation of AKT, NF-κB, and MAPK pathway and promoting insulin resistance [86].
Regarding its role in multiple myeloma, one case-control study showed that serum resistin was lower in MM patients (n = 73, 9.4 ± 5.0 ng/mL) compared to gender and age-matched healthy controls (n = 73, 15.9 ± 6.8 ng/mL, p < 0.001) [87]. A nested case-control study involving 178 MM patients and 358 controls showed lower resistin levels in male MM patients (5.2 (3.93–6.46) ng/mL) than controls (5.82 (4.44–7.33) ng/mL, p = 0.006) [88]. In contrast, using the primary myeloma cells and myeloma cell lines, Pang et al. have found that resistin suppressed caspase cleavage to promote drug resistance through the NF-κB and PI3K/Akt pathways. Besides, they demonstrated that resistin increased the expression of ATP-binding cassette (ABC) transporters and induced the ATP-driven efflux of chemotherapy drugs. In vivo mice model, they validated that resistin plays an anti-apoptosis anti-apoptotic role by annexin Ⅴ binding assay and in situ TUNEL assay [89]. Interestingly Reseland et al. showed that the level of resistin had no significant difference between the newly diagnosed MM patients and controls [49]. Pooled analysis of 367 MM patients and 524 controls showed no significant difference in circulating resistin levels (SMD = −0.08, 95%CI = −0.55 to 0.39, p = 0.73) [50].
Above all, the role of resistin in MM is polarized. Some studies demonstrated it is a protective adipokine; Pang et al. considered resistin is bad for MM through promoting drug resistance. More studies have shown no discrepancy in the level of resistin between MM patients and controls. The discrepancy in the role of resistin in MM could be explained as follows: First, the secretion of resistin is associated with the dietary approaches, BMI, and the level of glucose [90]. In vitro experiments were not affected by the above factors. Second, the different types, stages, and risk stratification may be responsible. However, the exact relationship between resistin and MM warrants further investigation.
Apelin
There are two ligands of apelin-angiotensin receptor-like1 (APJ). One is elabela/toddler (ELA), the other is apelin [91, 92]. Apelin also is an adipokine with several functions in many physiological and pathological processes, such as angiogenesis, fluid homeostasis, food intake, and metabolic regulation [93, 94]. The obese subjects presented a higher serum concentration of apelin than controls. The obese subjects (BMI ranged from 31 to 34 kg/m2) presented a higher serum concentration of apelin than age-matched controls (BMI ranged from 23 to 24 kg/m2) [95]. It was demonstrated that apelin had important effects on malignant diseases. The apelin and apelin receptor systems regulate autophagy and apoptosis [96–98]. Apelin was shown to be elevated (1.99 ± 1.1 ng/mL vs. 0.42 ± 0.16 ng/mL) in patients with MM (n = 29) when compared with healthy controls (n = 19). Furthermore, the level of apelin may associate with angiogenesis [99]. Some gains have been made in the mechanism of the affection of apelin in several cancers. The exact relationship between apelin and MM warrants further investigation. However, clinical studies with larger sample sizes are needed to support the correlation between MM and apelin.
Chemerin
Chemerin was first described as a chemoattractant agent, promoting the chemotaxis of leukocyte populations [100]. The highest expression of chemerin has been detected in white adipose tissue, liver, and lung [101]. Then chemerin is considered an adipokine involved in inflammation, adipogenesis, immunity, and energy metabolism [102]. A growing body of human experimental data indicated that serum chenerin chemerin levels are elevated in obese patients [103]. Increased chemerin levels have been found in gastric, colorectal, and pancreatic cancer [104–106]. The pro-tumor mechanism of chemerin is to promote the proliferation and migration of tumor cells [107, 108]. Westhrin et al. determined the serum levels of chemerin between MM patients (n = 122) and healthy controls (n = 58). They found chemerin serum levels of MM patients was higher than healthy controls (199.2 ± 88.2 ng/mL vs. 156.5 ± 52.5 ng/mL, p < 0.001). It was also shown that the levels of chemerin were associated with the stage of MM [109]. A study has shown that chemerin suppressed the differentiation of osteoblast [110]. It is not known whether chemerin can also play similar roles in myeloma bone disease. As a relatively new adipokine, data on chemerin involvement in MM are sparse. Nonetheless, the clinical evidence demonstrated that chemerin is increased in MM patients, which indicated that possibilities for chemerin as a biomarker should be further explored [109].
Conclusion
As MM is the second most common form of hematological malignancy and the incidence of obesity is increasing, the need for awareness of the association between obesity and MM development and progression is evident. The correlation between obesity and MM has gained significant attention in recent times. Emma V. Morris and Claire M. Edwards have discussed the association between adiposity, adipokines, and MM [111]. Our review includes new publications from the last 5 years that explore the relationship between MM and new adipokines, including apelin and chemerin. Furthermore, we have also included recent clinical studies that examine the levels of specific adipokines in MM patients, such as leptin. Additionally, we have delved into more in-depth investigations of the roles that visfatin and resistin play in MM. Lastly, the effect of adipokines on the immune microenvironment of MM is a new research highlight.
In this review, we have briefly summarized recent work on representative adipokines linked to MM initiation and progression (Table 2). First, the circulating levels of adipokines showed discrepancies when compared with control groups. Adipokines may be helpful as a biomarker for MM. In addition, adipokines such as adiponectin, leptin, and visfatin can regulate several aspects of myelosis, including promoting MM cell survival and creating a pro-tumorigenic environment for MM. Lastly, there is emerging evidence from in vitro studies that showed adipokines interventions could positively affect disease course.
However, steps forward are hampered by the variation in study outcomes, such as resistin hindered by the inconsistencies in study outcomes, such as resistin. The large heterogeneity partly explains such a phenomenon in MM patients. Based on these achievements, future work should better address as follows: (1) Conducting more clinical and cohorts researches on circulating adipokine levels and the correlation with known biomarkers of immunotypes, bone destruction, and progression. (2) The mechanisms of adipokines affect myelosis and elucidate the pathways of adipokines effects and underlying mechanism in the development of MM. (3) Find potential therapeutic implications target adipokines to halt MM. Taken together, adipokines are promising candidates both for novel pharmacological treatment strategies and as diagnostic tools, provided that we can develop a better understanding of the function and molecular targets of the more recently discovered adipokines.
Author contributions
WT: Writing-Original draft. LZ and LL: Funding acquisition. TM and ZY: Writing-Review and editing. JL: Visualization. YL and JB: Supervision. All authors contributed to the article and approved the submitted version.
Funding
This review was funded by National Clinical Medicine Research Center for Hematological System Diseases, grant number “2021WWA01,” the Talent Innovation and Entrepreneurshio Project of Lanzhou, grant number “2020-RC-48” and the Cuiying Scientific and Technological Innovation Program of Lanzhou University Second Hospital, grant number “CY2017-ZD04, CY2019-MS14.”
Conflict of interest
The authors declare that the research was conducted in the absence of any commercial or financial relationships that could be construed as a potential conflict of interest.
References
1. Cowan, AJ, Green, DJ, Kwok, M, Lee, S, Coffey, DG, Holmberg, LA, et al. Diagnosis and management of multiple myeloma: A review. JAMA (2022) 327:464–77. doi:10.1001/jama.2022.0003
2. van de Donk, NWCJ, Pawlyn, C, and Yong, KL. Multiple myeloma. Lancet (2021) 397:410–27. doi:10.1016/S0140-6736(21)00135-5
3. Podar, K, Richardson, PG, Hideshima, T, Chauhan, D, and Anderson, KC. The malignant clone and the bone-marrow environment. Best Pract Res Clin Haematol (2007) 20:597–612. doi:10.1016/j.beha.2007.08.002
4. Teras, LR, Kitahara, CM, Birmann, BM, Hartge, PA, Wang, SS, Robien, K, et al. Body size and multiple myeloma mortality: A pooled analysis of 20 prospective studies. Br J Haematol (2014) 166:667–76. doi:10.1111/bjh.12935
5. Kleinstern, G, Larson, DR, Allmer, C, Norman, AD, Muntifering, G, Sinnwell, J, et al. Body mass index associated with monoclonal gammopathy of undetermined significance (MGUS) progression in Olmsted County, Minnesota. Blood Cancer J (2022) 12:67. doi:10.1038/s41408-022-00659-9
6. Went, M, Cornish, AJ, Law, PJ, Kinnersley, B, van Duin, M, Weinhold, N, et al. Search for multiple myeloma risk factors using Mendelian randomization. Blood Adv (2020) 4:2172–9. doi:10.1182/bloodadvances.2020001502
7. Veldhuis-Vlug, AG, and Rosen, CJ. Clinical implications of bone marrow adiposity. J Intern Med (2018) 283:121–39. doi:10.1111/joim.12718
8. Morris, EV, Suchacki, KJ, Hocking, J, Cartwright, R, Sowman, A, Gamez, B, et al. Myeloma cells down-regulate adiponectin in bone marrow adipocytes via TNF-alpha. J Bone Mineral Res (2020) 35:942–55. doi:10.1002/jbmr.3951
9. Bullwinkle, EM, Parker, MD, Bonan, NF, Falkenberg, LG, Davison, SP, and DeCicco-Skinner, KL. Adipocytes contribute to the growth and progression of multiple myeloma: Unraveling obesity related differences in adipocyte signaling. Cancer Lett (2016) 380:114–21. doi:10.1016/j.canlet.2016.06.010
10. Lauby-Secretan, B, Scoccianti, C, Loomis, D, Grosse, Y, Bianchini, F, Straif, K, et al. Body fatness and cancer--viewpoint of the IARC working group. N Engl J Med (2016) 375:794–8. doi:10.1056/NEJMsr1606602
11. Avgerinos, KI, Spyrou, N, Mantzoros, CS, and Dalamaga, M. Obesity and cancer risk: Emerging biological mechanisms and perspectives. Metabolism (2019) 92:121–35. doi:10.1016/j.metabol.2018.11.001
12. Tarasiuk, A, Mosińska, P, and Fichna, J. The mechanisms linking obesity to colon cancer: An overview. Obes Res Clin Pract (2018) 12:251–9. doi:10.1016/j.orcp.2018.01.005
13. Divella, R, De Luca, R, Abbate, I, Naglieri, E, and Daniele, A. Obesity and cancer: The role of adipose tissue and adipo-cytokines-induced chronic inflammation. J Cancer (2016) 7:2346–59. doi:10.7150/jca.16884
14. Diedrich, J, Gusky, HC, and Podgorski, I. Adipose tissue dysfunction and its effects on tumor metabolism. Horm Mol Biol Clin Investig (2015) 21:17–41. doi:10.1515/hmbci-2014-0045
15. Vegiopoulos, A, Rohm, M, and Herzig, S. Adipose tissue: Between the extremes. EMBO J (2017) 36:1999–2017. doi:10.15252/embj.201696206
16. Fasshauer, M, and Blüher, M. Adipokines in health and disease. Trends Pharmacol Sci (2015) 36:461–70. doi:10.1016/j.tips.2015.04.014
17. Khandekar, MJ, Cohen, P, and Spiegelman, BM. Molecular mechanisms of cancer development in obesity. Nat Rev Cancer (2011) 11:886–95. doi:10.1038/nrc3174
18. Khoramipour, K, Chamari, K, Hekmatikar, AA, Ziyaiyan, A, Taherkhani, S, Elguindy, NM, et al. Adiponectin: Structure, physiological functions, role in diseases, and effects of nutrition. Nutrients (2021) 13:1180. doi:10.3390/nu13041180
19. Cawthorn, WP, Scheller, EL, Learman, BS, Parlee, SD, Simon, BR, Mori, H, et al. Bone marrow adipose tissue is an endocrine organ that contributes to increased circulating adiponectin during caloric restriction. Cell Metab (2014) 20:368–75. doi:10.1016/j.cmet.2014.06.003
20. Yamauchi, T, Iwabu, M, Okada-Iwabu, M, and Kadowaki, T. Adiponectin receptors: A review of their structure, function and how they work. Best Pract Res Clin Endocrinol Metab (2014) 28:15–23. doi:10.1016/j.beem.2013.09.003
21. Hug, C, Wang, J, Ahmad, NS, Bogan, JS, Tsao, T-S, and Lodish, HF. T-cadherin is a receptor for hexameric and high-molecular-weight forms of Acrp30/adiponectin. Proc Natl Acad Sci U. S. A. (2004) 101:10308–13. doi:10.1073/pnas.0403382101
22. Fang, H, and Judd, RL. Adiponectin regulation and function. Compr Physiol (2018) 8:1031–63. doi:10.1002/cphy.c170046
23. Lindberg, S, Jensen, JS, Pedersen, SH, Galatius, S, Frystyk, J, Flyvbjerg, A, et al. Low adiponectin levels and increased risk of type 2 diabetes in patients with myocardial infarction. Diabetes Care (2014) 37:3003–8. doi:10.2337/dc14-0932
24. Liu, L, Shi, Z, Ji, X, Zhang, W, Luan, J, Zahr, T, et al. Adipokines, adiposity, and atherosclerosis. Cell Mol Life Sci (2022) 79:272. doi:10.1007/s00018-022-04286-2
25. Yanai, H, and Yoshida, H. Beneficial effects of adiponectin on glucose and lipid metabolism and atherosclerotic progression: Mechanisms and perspectives. Int J Mol Sci (2019) 20:1190. doi:10.3390/ijms20051190
26. Ma, W, Zhang, S, Li, Y, Chen, T, Yang, Q, and Feng, X. Adiponectin alleviates nonalcoholic fatty liver injury via regulating oxidative stress in liver cells. Minerva Med (2020) 113:990–9. doi:10.23736/S0026-4806.20.06734-8
27. Tilg, H, and Moschen, AR. Adipocytokines: Mediators linking adipose tissue, inflammation and immunity. Nat Rev Immunol (2006) 6:772–83. doi:10.1038/nri1937
28. Di Zazzo, E, Polito, R, Bartollino, S, Nigro, E, Porcile, C, Bianco, A, et al. Adiponectin as link factor between adipose tissue and cancer. Int J Mol Sci (2019) 20:839. doi:10.3390/ijms20040839
29. Hofmann, JN, Mailankody, S, Korde, N, Wang, Y, Tageja, N, Costello, R, et al. Circulating adiponectin levels differ between patients with multiple myeloma and its precursor disease. Obesity (Silver Spring) (2017) 25:1317–20. doi:10.1002/oby.21894
30. Hofmann, JN, Birmann, BM, Teras, LR, Pfeiffer, RM, Wang, Y, Albanes, D, et al. Low levels of circulating adiponectin are associated with multiple myeloma risk in overweight and obese individuals. Cancer Res (2016) 76:1935–41. doi:10.1158/0008-5472.CAN-15-2406
31. Fowler, JA, Lwin, ST, Drake, MT, Edwards, JR, Kyle, RA, Mundy, GR, et al. Host-derived adiponectin is tumor-suppressive and a novel therapeutic target for multiple myeloma and the associated bone disease. Blood (2011) 118:5872–82. doi:10.1182/blood-2011-01-330407
32. Medina, EA, Oberheu, K, Polusani, SR, Ortega, V, Velagaleti, GVN, and Oyajobi, BO. PKA/AMPK signaling in relation to adiponectin's antiproliferative effect on multiple myeloma cells. Leukemia (2014) 28:2080–9. doi:10.1038/leu.2014.112
33. Akhmetzyanova, I, Aaron, T, Galbo, P, Tikhonova, A, Dolgalev, I, Tanaka, M, et al. Tissue-resident macrophages promote early dissemination of multiple myeloma via IL-6 and TNFα. Blood Adv (2021) 5:3592–608. doi:10.1182/bloodadvances.2021005327
34. Meacham, CE, Jeffery, EC, Burgess, RJ, Sivakumar, CD, Arora, MA, Stanley, AM, et al. Adiponectin receptors sustain haematopoietic stem cells throughout adulthood by protecting them from inflammation. Nat Cell Biol (2022) 24:697–707. doi:10.1038/s41556-022-00909-9
35. Bernstein, ZS, Kim, EB, and Raje, N. Bone disease in multiple myeloma: Biologic and clinical implications. Cells (2022) 11:2308. doi:10.3390/cells11152308
36. Liu, Z, Liu, H, Li, Y, Wang, Y, Xing, R, Mi, F, et al. Adiponectin inhibits the differentiation and maturation of osteoclasts via the mTOR pathway in multiple myeloma. Int J Mol Med (2020) 45:1112–20. doi:10.3892/ijmm.2020.4475
37. Olechnowicz, SWZ, Weivoda, MM, Lwin, ST, Leung, SK, Gooding, S, Nador, G, et al. Multiple myeloma increases nerve growth factor and other pain-related markers through interactions with the bone microenvironment. Scientific Rep (2019) 9:14189. doi:10.1038/s41598-019-50591-5
38. Peterson, SJ, Drummond, G, Kim, DH, Li, M, Kruger, AL, Ikehara, S, et al. L-4F treatment reduces adiposity, increases adiponectin levels, and improves insulin sensitivity in obese mice. J Lipid Res (2008) 49:1658–69. doi:10.1194/jlr.M800046-JLR200
39. Zhang, Y, Proenca, R, Maffei, M, Barone, M, Leopold, L, and Friedman, JM. Positional cloning of the mouse obese gene and its human homologue. Nature (1994) 372:425–32. doi:10.1038/372425a0
40. Trinh, T, and Broxmeyer, HE. Role for leptin and leptin receptors in stem cells during health and diseases. Stem Cell Rev Rep (2021) 17:511–22. doi:10.1007/s12015-021-10132-y
41. Obradovic, M, Sudar-Milovanovic, E, Soskic, S, Essack, M, Arya, S, Stewart, AJ, et al. Leptin and obesity: Role and clinical implication. Front Endocrinol (2021) 12:585887. doi:10.3389/fendo.2021.585887
42. Considine, RV, Sinha, MK, Heiman, ML, Kriauciunas, A, Stephens, TW, Nyce, MR, et al. Serum immunoreactive-leptin concentrations in normal-weight and obese humans. N Engl J Med (1996) 334:292–5. doi:10.1056/NEJM199602013340503
43. Mhaidat, NM, Alzoubi, KH, Kubas, MA, Banihani, MN, Hamdan, N, and Al-Jaberi, TM. High levels of leptin and non-high molecular weight-adiponectin in patients with colorectal cancer: Association with chemotherapy and common genetic polymorphisms. Biomed Rep (2021) 14:13. doi:10.3892/br.2020.1389
44. García-Estevez, L, González-Martínez, S, and Moreno-Bueno, G. The leptin Axis and its association with the adaptive immune system in breast cancer. Front Immunol (2021) 12:784823. doi:10.3389/fimmu.2021.784823
45. Xu, CJ, Dong, LL, Kang, XL, Li, ZM, and Zhang, HY. Leptin promotes proliferation and inhibits apoptosis of prostate cancer cells by regulating ERK1/2 signaling pathway. Eur Rev Med Pharmacol Sci (2020) 24:8341–8. doi:10.26355/eurrev_202008_22630
46. Wang, J, Zhou, F, Li, F, Wang, B, Hu, Y, and Li, X. Autocrined leptin promotes proliferation of non-small cell lung cancer (NSCLC) via PI3K/AKT and p53 pathways. Ann Transl Med (2021) 9:568. doi:10.21037/atm-20-7482
47. Kashiwagi, E, Abe, T, Kinoshita, F, Ushijima, M, Masaoka, H, Shiota, M, et al. The role of adipocytokines and their receptors in bladder cancer: Expression of adiponectin or leptin is an independent prognosticator. Am J Transl Res (2020) 12:e195–45. doi:10.1016/s2666-1683(20)32677-x
48. Pamuk, GE, Demir, M, Harmandar, F, Yesil, Y, Turgut, B, and Vural, O. Leptin and resistin levels in serum of patients with hematologic malignancies: Correlation with clinical characteristics. Exp Oncol (2006) 28:241–4.
49. Reseland, JE, Reppe, S, Olstad, OK, Hjorth-Hansen, H, Brenne, AT, Syversen, U, et al. Abnormal adipokine levels and leptin-induced changes in gene expression profiles in multiple myeloma. Eur J Haematol (2009) 83:460–70. doi:10.1111/j.1600-0609.2009.01311.x
50. Liu, R, Gao, D, Lv, Y, Zhai, M, and He, A. Importance of circulating adipocytokines in multiple myeloma: A systematic review and meta-analysis based on case-control studies. BMC Endocr Disord (2022) 22:29. doi:10.1186/s12902-022-00939-2
51. Alexandrakis, MG, Passam, FH, Sfiridaki, A, Pappa, CA, Moschandrea, JA, Kandidakis, E, et al. Serum levels of leptin in multiple myeloma patients and its relation to angiogenic and inflammatory cytokines. The Int J Biol Markers (2004) 19:52–7. doi:10.5301/jbm.2008.510
52. Hofmann, JN, Liao, LM, Pollak, MN, Wang, Y, Pfeiffer, RM, Baris, D, et al. A prospective study of circulating adipokine levels and risk of multiple myeloma. Blood (2012) 120:4418–20. doi:10.1182/blood-2012-06-438606
53. Yu, W, Cao, D-D, Li, Q-B, Mei, H-L, Hu, Y, and Guo, T. Adipocytes secreted leptin is a pro-tumor factor for survival of multiple myeloma under chemotherapy. Oncotarget (2016) 7:86075–86. doi:10.18632/oncotarget.13342
54. Liu, Z, Xu, J, He, J, Liu, H, Lin, P, Wan, X, et al. Mature adipocytes in bone marrow protect myeloma cells against chemotherapy through autophagy activation. Oncotarget (2015) 6:34329–41. doi:10.18632/oncotarget.6020
55. Chan, AC, Neeson, P, Leeansyah, E, Tainton, K, Quach, H, Prince, HM, et al. Natural killer T cell defects in multiple myeloma and the impact of lenalidomide therapy. Clin Exp Immunol (2014) 175:49–58. doi:10.1111/cei.12196
56. Dhodapkar, MV, and Richter, J. Harnessing natural killer T (NKT) cells in human myeloma: Progress and challenges. Clin Immunol (Orlando, Fla (2011) 140:160–6. doi:10.1016/j.clim.2010.12.010
57. Favreau, M, Menu, E, Gaublomme, D, Vanderkerken, K, Faict, S, Maes, K, et al. Leptin receptor antagonism of iNKT cell function: A novel strategy to combat multiple myeloma. Leukemia (2017) 31:2678–85. doi:10.1038/leu.2017.146
58. Wang, Z, Aguilar, EG, Luna, JI, Dunai, C, Khuat, LT, Le, CT, et al. Paradoxical effects of obesity on T cell function during tumor progression and PD-1 checkpoint blockade. Nat Med (2019) 25:141–51. doi:10.1038/s41591-018-0221-5
59. Sih, R, Morley, JE, Kaiser, FE, Perry, HM, Patrick, P, and Ross, C. Testosterone replacement in older hypogonadal men: A 12-month randomized controlled trial. J Clin Endocrinol Metab (1997) 82:1661–7. doi:10.1210/jcem.82.6.3988
60. Simon, C, Gronfier, C, Schlienger, JL, and Brandenberger, G. Circadian and ultradian variations of leptin in normal man under continuous enteral nutrition: Relationship to sleep and body temperature. J Clin Endocrinol Metab (1998) 83:1893–9. doi:10.1210/jcem.83.6.4864
61. Montague, CT, Prins, JB, Sanders, L, Digby, JE, and O'Rahilly, S. Depot- and sex-specific differences in human leptin mRNA expression: Implications for the control of regional fat distribution. Diabetes (1997) 46:342–7. doi:10.2337/diab.46.3.342
62. Samal, B, Sun, Y, Stearns, G, Xie, C, Suggs, S, and McNiece, I. Cloning and characterization of the cDNA encoding a novel human pre-B-cell colony-enhancing factor. Mol Cell Biol (1994) 14:1431–7. doi:10.1128/mcb.14.2.1431
63. Zhai, RG, Rizzi, M, and Garavaglia, S. Nicotinamide/nicotinic acid mononucleotide adenylyltransferase, new insights into an ancient enzyme. Cell Mol Life Sci (2009) 66:2805–18. doi:10.1007/s00018-009-0047-x
64. Fukuhara, A, Matsuda, M, Nishizawa, M, Segawa, K, Tanaka, M, Kishimoto, K, et al. Visfatin: A protein secreted by visceral fat that mimics the effects of insulin. Science (New York, N.Y.) (2005) 307:426–30. doi:10.1126/science.1097243
65. Chang, Y-H, Chang, D-M, Lin, K-C, Shin, S-J, and Lee, Y-J. Visfatin in overweight/obesity, type 2 diabetes mellitus, insulin resistance, metabolic syndrome and cardiovascular diseases: A meta-analysis and systemic review. Diabetes Metab Res Rev (2011) 27:515–27. doi:10.1002/dmrr.1201
66. Fazeli, MS, Dashti, H, Akbarzadeh, S, Assadi, M, Aminian, A, Keramati, MR, et al. Circulating levels of novel adipocytokines in patients with colorectal cancer. Cytokine (2013) 62:81–5. doi:10.1016/j.cyto.2013.02.012
67. Nakajima, TE, Yamada, Y, Hamano, T, Furuta, K, Gotoda, T, Katai, H, et al. Adipocytokine levels in gastric cancer patients: Resistin and visfatin as biomarkers of gastric cancer. J Gastroenterol (2009) 44:685–90. doi:10.1007/s00535-009-0063-5
68. Reddy, PS, Umesh, S, Thota, B, Tandon, A, Pandey, P, Hegde, AS, et al. PBEF1/NAmPRTase/Visfatin: A potential malignant astrocytoma/glioblastoma serum marker with prognostic value. Cancer Biol Ther (2008) 7:663–8. doi:10.4161/cbt.7.5.5663
69. Lu, Z, Fang, Z, Guo, Y, Liu, X, and Chen, S. Cisplatin resistance of NSCLC cells involves upregulation of visfatin through activation of its transcription and stabilization of mRNA. Chem Biol Interact (2022) 351:109705:109705. doi:10.1016/j.cbi.2021.109705
70. Bong, IPN, Ng, CC, Fakiruddin, SK, Lim, MN, and Zakaria, Z. Small interfering RNA-mediated silencing of nicotinamide phosphoribosyltransferase (NAMPT) and lysosomal trafficking regulator (lyst) induce growth inhibition and apoptosis in human multiple myeloma cells: A preliminary study. Bosnian J Basic Med Sci (2016) 16:268–75. doi:10.17305/bjbms.2016.1568
71. Cea, M, Cagnetta, A, Patrone, F, Nencioni, A, Gobbi, M, and Anderson, KC. Intracellular NAD(+) depletion induces autophagic death in multiple myeloma cells. Autophagy (2013) 9:410–2. doi:10.4161/auto.22866
72. Cea, M, Cagnetta, A, Fulciniti, M, Tai, Y-T, Hideshima, T, Chauhan, D, et al. Targeting NAD+ salvage pathway induces autophagy in multiple myeloma cells via mTORC1 and extracellular signal-regulated kinase (ERK1/2) inhibition. Blood (2012) 120:3519–29. doi:10.1182/blood-2012-03-416776
73. Cagnetta, A, Cea, M, Calimeri, T, Acharya, C, Fulciniti, M, Tai, Y-T, et al. Intracellular NAD⁺ depletion enhances bortezomib-induced anti-myeloma activity. Blood (2013) 122:1243–55. doi:10.1182/blood-2013-02-483511
74. Kennedy, BE, Sadek, M, Elnenaei, MO, Reiman, A, and Gujar, SA. Targeting NAD+ synthesis to potentiate CD38-based immunotherapy of multiple myeloma. Trends Cancer (2020) 6:9–12. doi:10.1016/j.trecan.2019.11.005
75. Venkateshaiah, SU, Khan, S, Ling, W, Bam, R, Li, X, van Rhee, F, et al. NAMPT/PBEF1 enzymatic activity is indispensable for myeloma cell growth and osteoclast activity. Exp Hematol (2013) 41:547–57. doi:10.1016/j.exphem.2013.02.008
76. Steppan, CM, Bailey, ST, Bhat, S, Brown, EJ, Banerjee, RR, Wright, CM, et al. The hormone resistin links obesity to diabetes. Nature (2001) 409:307–12. doi:10.1038/35053000
77. Patel, L, Buckels, AC, Kinghorn, IJ, Murdock, PR, Holbrook, JD, Plumpton, C, et al. Resistin is expressed in human macrophages and directly regulated by PPAR gamma activators. Biochem Biophys Res Commun (2003) 300:472–6. doi:10.1016/s0006-291x(02)02841-3
78. Deb, A, Deshmukh, B, Ramteke, P, Bhati, FK, and Bhat, MK. Resistin: A journey from metabolism to cancer. Transl Oncol (2021) 14:101178. doi:10.1016/j.tranon.2021.101178
79. Qiu, L, Zhang, G-F, Yu, L, Wang, H-Y, Jia, X-J, and Wang, T-J. Novel oncogenic and chemoresistance-inducing functions of resistin in ovarian cancer cells require miRNAs-mediated induction of epithelial-to-mesenchymal transition. Scientific Rep (2018) 8:12522. doi:10.1038/s41598-018-30978-6
80. Lee, JO, Kim, N, Lee, HJ, Lee, YW, Kim, SJ, Park, SH, et al. Resistin, a fat-derived secretory factor, promotes metastasis of MDA-MB-231 human breast cancer cells through ERM activation. Scientific Rep (2016) 6:18923. doi:10.1038/srep18923
81. Pang, L, Zhang, Y, Yu, Y, and Zhang, S. Resistin promotes the expression of vascular endothelial growth factor in ovary carcinoma cells. Int J Mol Sci (2013) 14:9751–66. doi:10.3390/ijms14059751
82. Rak, A, Drwal, E, Wróbel, A, and Gregoraszczuk, EŁ. Resistin is a survival factor for porcine ovarian follicular cells. Reproduction (Cambridge, England) (2015) 150:343–55. doi:10.1530/REP-15-0255
83. Mu, H, Ohashi, R, Yan, S, Chai, H, Yang, H, Lin, P, et al. Adipokine resistin promotes in vitro angiogenesis of human endothelial cells. Cardiovasc Res (2006) 70:146–57. doi:10.1016/j.cardiores.2006.01.015
84. Owecki, M, Miczke, A, Nikisch, E, Pupek-Musialik, D, and Sowiński, J. Serum resistin concentrations are higher in human obesity but independent from insulin resistance. Exp Clin Endocrinol Diabetes (2011) 119:117–21. doi:10.1055/s-0030-1263111
85. Deshmukh, SK, Srivastava, SK, Zubair, H, Bhardwaj, A, Tyagi, N, Al-Ghadhban, A, et al. Resistin potentiates chemoresistance and stemness of breast cancer cells: Implications for racially disparate therapeutic outcomes. Cancer Lett (2017) 396:21–9. doi:10.1016/j.canlet.2017.03.010
86. Benomar, Y, Gertler, A, De Lacy, P, Crépin, D, Ould Hamouda, H, Riffault, L, et al. Central resistin overexposure induces insulin resistance through Toll-like receptor 4. Diabetes (2013) 62:102–14. doi:10.2337/db12-0237
87. Dalamaga, M, Karmaniolas, K, Panagiotou, A, Hsi, A, Chamberland, J, Dimas, C, et al. Low circulating adiponectin and resistin, but not leptin, levels are associated with multiple myeloma risk: A case-control study. Cancer Causes Control (2009) 20:193–9. doi:10.1007/s10552-008-9233-7
88. Santo, L, Teras, LR, Giles, GG, Weinstein, SJ, Albanes, D, Wang, Y, et al. Circulating resistin levels and risk of multiple myeloma in three prospective cohorts. Br J Cancer (2017) 117:1241–5. doi:10.1038/bjc.2017.282
89. Pang, J, Shi, Q, Liu, Z, He, J, Liu, H, Lin, P, et al. Resistin induces multidrug resistance in myeloma by inhibiting cell death and upregulating ABC transporter expression. Haematologica (2017) 102:1273–80. doi:10.3324/haematol.2016.154062
90. Millar, SR, Navarro, P, Harrington, JM, Shivappa, N, Hébert, JR, Perry, IJ, et al. Dietary score associations with markers of chronic low-grade inflammation: A cross-sectional comparative analysis of a middle-to older-aged population. Eur J Nutr (2022) 61:3377–90. doi:10.1007/s00394-022-02892-1
91. Tatemoto, K, Hosoya, M, Habata, Y, Fujii, R, Kakegawa, T, Zou, MX, et al. Isolation and characterization of a novel endogenous peptide ligand for the human APJ receptor. Biochem Biophys Res Commun (1998) 251:471–6. doi:10.1006/bbrc.1998.9489
92. Charo, DN, Ho, M, Fajardo, G, Kawana, M, Kundu, RK, Sheikh, AY, et al. Endogenous regulation of cardiovascular function by apelin-APJ. Am J Physiol Heart Circulatory Physiol (2009) 297:H1904–13. doi:10.1152/ajpheart.00686.2009
93. Kershaw, EE, and Flier, JS. Adipose tissue as an endocrine organ. J Clin Endocrinol Metab (2004) 89:2548–56. doi:10.1210/jc.2004-0395
94. Dawid, M, Mlyczyńska, E, Jurek, M, Respekta, N, Pich, K, Kurowska, P, et al. Apelin, APJ, and ELABELA: Role in placental function, pregnancy, and foetal development-an overview. Cells (2021) 11:99. doi:10.3390/cells11010099
95. Boucher, J, Masri, B, Daviaud, D, Gesta, S, Guigné, C, Mazzucotelli, A, et al. Apelin, a newly identified adipokine up-regulated by insulin and obesity. Endocrinology (2005) 146:1764–71. doi:10.1210/en.2004-1427
96. Picault, F-X, Chaves-Almagro, C, Projetti, F, Prats, H, Masri, B, and Audigier, Y. Tumour co-expression of apelin and its receptor is the basis of an autocrine loop involved in the growth of colon adenocarcinomas. Eur J Cancer (2014) 50:663–74. doi:10.1016/j.ejca.2013.11.017
97. Sorli, SC, Le Gonidec, S, Knibiehler, B, and Audigier, Y. Apelin is a potent activator of tumour neoangiogenesis. Oncogene (2007) 26:7692–9. doi:10.1038/sj.onc.1210573
98. Kälin, RE, and Glass, R. APLN/APLNR signaling controls key pathological parameters of glioblastoma. Cancers (2021) 13:3899. doi:10.3390/cancers13153899
99. Maden, M, Pamuk, ON, and Pamuk, GE. High apelin levels could be used as a diagnostic marker in multiple myeloma: A comparative study. Cancer Biomark (2016) 17:391–6. doi:10.3233/CBM-160654
100. Fischer, TF, and Beck-Sickinger, AG. Chemerin - exploring a versatile adipokine. Biol Chem (2022) 403:625–42. doi:10.1515/hsz-2021-0409
101. Helfer, G, and Wu, Q-F. Chemerin: A multifaceted adipokine involved in metabolic disorders. J Endocrinol (2018) 238:R79–R94. doi:10.1530/JOE-18-0174
102. Bozaoglu, K, Curran, JE, Stocker, CJ, Zaibi, MS, Segal, D, Konstantopoulos, N, et al. Chemerin, a novel adipokine in the regulation of angiogenesis. J Clin Endocrinol Metab (2010) 95:2476–85. doi:10.1210/jc.2010-0042
103. Rourke, JL, Dranse, HJ, and Sinal, CJ. Towards an integrative approach to understanding the role of chemerin in human health and disease. Obes Rev (2013) 14:245–62. doi:10.1111/obr.12009
104. Wang, C, Wu, WKK, Liu, X, To, K-F, Chen, GG, Yu, J, et al. Increased serum chemerin level promotes cellular invasiveness in gastric cancer: A clinical and experimental study. Peptides (2014) 51:131–8. doi:10.1016/j.peptides.2013.10.009
105. Erdogan, S, Yilmaz, FM, Yazici, O, Yozgat, A, Sezer, S, Ozdemir, N, et al. Inflammation and chemerin in colorectal cancer. Tumour Biol (2016) 37:6337–42. doi:10.1007/s13277-015-4483-y
106. Wang, Q, Wang, H, Ding, Y, Wan, M, and Xu, M. The role of adipokines in pancreatic cancer. Front Oncol (2022) 12:926230. doi:10.3389/fonc.2022.926230
107. Gao, C, Shi, J, Zhang, J, Li, Y, and Zhang, Y. Chemerin promotes proliferation and migration of ovarian cancer cells by upregulating expression of PD-L1. J Zhejiang Univ Sci B (2022) 23:164–70. doi:10.1631/jzus.B2100392
108. Friebus-Kardash, J, Schulz, P, Reinicke, S, Karthaus, C, Schefer, Q, Bandholtz, S, et al. A chemerin peptide analog stimulates tumor growth in two xenograft mouse models of human colorectal carcinoma. Cancers (2021) 14:125. doi:10.3390/cancers14010125
109. Westhrin, M, Moen, SH, Kristensen, IB, Buene, G, Mylin, AK, Turesson, I, et al. Chemerin is elevated in multiple myeloma patients and is expressed by stromal cells and pre-adipocytes. Biomark Res (2018) 6:21. doi:10.1186/s40364-018-0134-y
110. Muruganandan, S, Roman, AA, and Sinal, CJ. Role of chemerin/CMKLR1 signaling in adipogenesis and osteoblastogenesis of bone marrow stem cells. J Bone Mineral Res (2010) 25:222–34. doi:10.1359/jbmr.091106
Keywords: multiple myeloma, adipokine, obesity, adiponectin, leptin
Citation: Tie W, Ma T, Yi Z, Liu J, Li Y, Bai J, Li L and Zhang L (2023) Obesity as a risk factor for multiple myeloma: insight on the role of adipokines. Pathol. Oncol. Res. 29:1611338. doi: 10.3389/pore.2023.1611338
Received: 05 June 2023; Accepted: 01 August 2023;
Published: 10 August 2023.
Edited by:
Anna Sebestyén, Semmelweis University, HungaryCopyright © 2023 Tie, Ma, Yi, Liu, Li, Bai, Li and Zhang. This is an open-access article distributed under the terms of the Creative Commons Attribution License (CC BY). The use, distribution or reproduction in other forums is permitted, provided the original author(s) and the copyright owner(s) are credited and that the original publication in this journal is cited, in accordance with accepted academic practice. No use, distribution or reproduction is permitted which does not comply with these terms.
*Correspondence: Lijuan Li, lijuan@sina.com; Liansheng Zhang, doctorzhanglsh@sina.com